Photonic Frontiers: Spectrometers and Spectroscopy: Looking Back/Looking Forward: Spectrometers and spectroscopy get faster, better, and cheaper
Faster, better, cheaper was former NASA administrator Dan Goldin’s mantra for improving space missions in the 1990s, but it might better describe the development of spectroscopic instruments over the past half-century. The concept of spectral measurement dates back to the 19th century, but spectroscopy was largely an academic matter well into the 20th century. Arnold Beckman’s commercial introduction of the DU Spectrophotometer in 1941 marked a commercial milestone—a product capable that could measure visible and ultraviolet spectra in a matter of minutes, making spectroscopy useful in the industrial laboratory.
Spectroscopy and spectrometers have come a long way.
Laser excitation and dye lasers
Early optical spectrometers depended on broadband light sources, but microwave spectroscopy used narrowband sources that could be tuned across the spectrum. Arthur Schawlow, who had studied both optical and microwave spectroscopy, saw the appeal of a tunable narrowband optical source when he was developing the laser. But early lasers had narrow gain bands, limiting the tuning range, even when applying external magnetic fields or changing the temperature.
In its early years, Laser Focus covered only lasers, so our interest in spectroscopy was limited. Our April 1, 1965 issue mentioned only three things described at the previous month’s Pittsburgh Conference on Analytical Chemistry and Applied Spectroscopy. One was a solid-state laser microprobe built by Jarrell Ash to excite line emission. The other two were Raman spectrometers that used gas lasers to excite Raman emission, made by Perkin-Elmer and the Applied Physics Corporation.
The first broadly tunable laser was not demonstrated until 1967, as we reported in July 1967. It took several steps to achieve, starting on February 7, 1966, when Peter Sorokin produced laser pulses by pumping an organic dye with a big ruby laser at the IBM Watson Research Center. Then, Mary Spaeth changed the wavelength of a dye laser between 761 and 789 nm by changing dye cells and mirror spacings at Hughes Aircraft Co. (Culver City, CA) Shortly after that, Fritz Schaefer at the Max Planck Institute in Germany showed emission wavelength changed with dye concentration. Next, Sorokin pumped a dye laser with a flashlamp (see Fig. 1), allowing the use of more wavelengths. Finally, in mid-1967, Bernard Soffer and Bill McFarland at Korad (Santa Monica, CA) replaced one cavity mirror with an adjustable diffraction grating, allowing continuous tuning of rhodamine 6 (in alcohol) across 20 nm.
The continuous-wave (CW) dye laser followed in 1970 after Ben Snavely, Otis Peterson, and Sam Tuccio at Eastman Kodak pumped a flowing dye solution with 1 W from an argon laser to produce 30 mW at 597 nm. The CW dye offered more precise spectroscopy, and stimulated a burst of interest in photochemistry. Our August 1974 issue quoted Richard Zare, then at Columbia University, as saying "We have in lasers an immensely powerful analytic tool which will revolutionized the detection of molecules." The hottest topic in photochemistry was laser enrichment of isotopes, particularly uranium isotopes for nuclear energy, reported at the International Quantum Electronics Conference in June (August 1974). Our IQEC report also described a new approach to high-resolution laser spectroscopy, demonstrated by Ken Nill at the MIT Lincoln Laboratory using cryogenically cooled tunable lead-tin-telluride diode lasers emitting up to 10 mW at 8.2–10.6 μm. By mid-1975, the Lawrence Livermore National Laboratory reported enriching macroscopic quantities of uranium (see Fig. 2).Laser and nonlinear spectrometers
A panel at SPIE’s 1974 annual meeting in San Diego saw dramatic scientific possibilities for laser spectroscopy, but was skeptical about its economics (October 1974). "With a good, small, cheap laser," said Stanley Klainer of Block Engineering, "we could build a remote monitoring system which will be very competitive." But he considered infrared spectrometers to be more highly developed, and lasers to be costly and inefficient. "What can the laser spectrometer do that the infrared spectrometer cannot do, and that would justify somebody spending [an additional] $50,000?" He concluded that laser spectroscopy would remain limited to special problems until lasers could be made cheaper and better.
By the mid-1970s, non-laser spectrometers had crept into ads for optical measurement instruments. Gamma Scientific (San Diego, CA) listed spectroradiometers among its measurement instruments (July 1974). But through the 1970s and 1980s, we focused more on the fascinating physics of laser spectroscopy and the large government program in laser uranium enrichment.
The growth of instrumentation
By 1990, spectroscopic instrumentation had become a very broad and sophisticated field, aided by advances in computer technology, and we were covering it more extensively. "Spectrometers have been computer-controlled for years. Within the last year or so, spectrometer manufacturers have pushed forward standardizing the computers used for analyzing data," we wrote (February 1990). IBM PCs and compatibles had taken over from an earlier generation of equipment that had used Apple II computers, and software was becoming faster, more flexible, and easier to use.
"For many routine applications, lasers are less useful than other sources of radiation" for spectrometers, wrote Bruce Hudson of the University of Oregon (Eugene, OR) in a February 1990 review. Laser spectroscopy had become important in research and diagnostic applications, but they still were rarely packaged into spectrometers other than as light sources for Raman spectroscopy or for pulsed excitation of emission spectra. Hudson noted that lasers were primarily being used for "entirely new methods of spectroscopy rather than incremental improvements in existing methods."
By 1995, spectrometers were shrinking in size and price. In March 1995, we reported that Ocean Optics was introducing a compact fiber-coupled spectrometer with up to a dozen diffraction gratings available for customization. Built around lead-sulfide detector arrays with 256 or 512 elements, it covered 1–3 μm for applications including telecommunications, medicine, and process monitoring. A separate Ocean Optics ad said prices started at $1800.LEDs, already used in special-purpose instruments such as blood oxygen monitors and having had their emission range expand to 2 μm, were opening the door to new types of compact spectrometers. A March 1995 feature (see Fig. 3) described how LED arrays made possible a near-infrared Fourier-transform spectrometer with no moving parts. When individual LEDs were modulated at different frequencies and focused on common detector, a standard Fourier transform could recover the modulated signals from each LED element, engineers from D.O.M. Associates (Manhattan, KS) reported.
Combining indium-gallium-arsenide detector arrays with imaging spectrographs makes near-infrared spectroscopic analysis faster and easier, a team from JY Horiba (Edison, NJ) reported in our May 2000 issue (see Fig. 4). Typical spectrometers use linear arrays of 128 to 512 elements, with dedicated software coordinating the spectrograph with the array. Cooling the detector array improves performance for low-light-level applications including photoluminescence measurements and Raman spectroscopy.Femtosecond laser pulses can generate ultrashort bursts of terahertz radiation, which can be focused onto samples for terahertz spectroscopy, Pengyu Han and Xi-Cheng Zhang of the Rensselaer Polytechnic Institute (Troy, NY) wrote in our October 2000 issue. The band is important because many biological and organic compounds have distinct terahertz signatures, important for applications ranging from medical imaging to spotting explosives. With several groups operating research systems, commercial equipment was expected "in the near future."
The incredible shrinking spectrometer
"Only in the last decade or so has spectroscopic instrumentation escaped the confines of the laboratory," wrote Rob Morris of Ocean Optics (Dunedin, FL) in our February 2001 issue. He credited mass production of fiber optics, improved efficiency and reduced cost of optics, and the digital revolution in expanding spectroscopic applications "into the field, into the factory, and even into the human body." With more than 50 types of spectrometers in commercial use, he put the total market for spectrometry and related optical sensors at billions of dollars.
Spectrometers have been shrinking in size as well as cost. In the early 1990s, he wrote, Ocean Optics introduced "a CCD array spectrometer small enough to fit inside a PC, and portable enough to bring the sensor to the sample." By 2001, more than a dozen companies offered miniature fiber-optic spectrometers, including one from Optical Coating Laboratory (Santa Rosa, CA) smaller than a credit card. The flexible and compact designs allow such applications as field studies of how the color of lizard skins affected the animals’ socialization (see Fig. 5).Imaging spectrometers able to record high-resolution spectra of every pixel "have been around for more than 20 years, enabling advances in environmental monitoring, defense and security, and even medical imaging," wrote John Kerekes of the Rochester Institute of Technology in our July 2006 issue. But he counted over a dozen companies that had begun making commercial versions for airborne imaging or for field or laboratory use. Noting the lack of "killer apps" in environmental monitoring or remote sensing, he suggested that industrial or medical applications might be the best prospects for widespread use.
Those trends benefited from the rising production volume and declining cost of lasers and optical products. "Today’s spectrometers are modern-day ‘tricorders,’ helping warehouse operators speed up identification and certification of incoming materials, detecting counterfeit drugs at border crossings, identifying possible bioweapons in airports or on the battlefield, and assisting first responders and firefighters with investigating suspicious substances or chemical spills," said Eric Bergles of BaySpec (San Jose, CA) in our August 2010 issue.
In September 2010, we described a novel compact spectrometer from Block Engineering (Marlborough, MA) based on tunable quantum-cascade lasers operating in the crucial mid-infrared band. It was designed to replace Fourier-transform infrared spectrometers for applications including military sensing, chemical and pharmaceutical manufacture, biomedical measurement, and environmental monitoring.
Miniature spectrometers small enough to hold in your hand are replacing bench spectrometers for many applications, Robert Chimenti of B&W Tek wrote in the May 2013 issue. Many are highly customized for quick identification by first responders. B&W Tek makes a narcotic-detection Raman spectrometer that includes a library of nearly 1000 narcotics, pharmaceuticals, cutting agents, and precursors.
Imaging spectrometers have become strikingly diverse, ranging from microimaging of biological specimens to remote sensing from satellites, senior editor John Wallace wrote in a January 2014 feature. Examples included nondestructive analysis of museum art and identification of diseased and healthy poultry.
Looking forward
As I write, the New Horizons spacecraft is slowly transmitting data from its Pluto flyby collected by its imaging spectrometers. Half a century ago, Pluto was just a speck in the sky to our best telescopes, but the instruments on New Horizons reveal it as a world, and spectroscopy tells us its mountains are made of ice.
Those instruments are harbingers of future spectroscopic instruments that will search for signs of life in the atmospheres of planets beyond the solar system. Astronomers have confirmed nearly 1600 exoplanets outside the solar systems, and planetary scientists are designing new instruments to explore them.
On the other end of the scale, spectrometers are coming closer to being everyday products. As in instruments for first responders, the spectroscopy will be hidden inside, with computer-driven interfaces to interpret observations for users. Yet the new instruments will not be all-purpose instruments like the fictional Star Trek Tricorder. We are far from having the technology needed to identify all possible materials in all possible environments.
Instead, new instruments may be able to switch among many libraries of characteristic spectra for various environments. A medical spectrometer might compare data records with one library for blood tests, another library for skin, and a third library for the interior of the eye. Such libraries could be used like apps on a smartphone. A household spectrometer could use one app to check foods for nut allergens, and another to check for traces of pathogens on food surfaces. Other apps could be developed to select wines, or to identify the plastic-wrapped mystery meat in the back of your freezer.
That’s only a sampling of the possibilities as the economies of scale drive down costs. We won’t see everything that we can imagine, but we will see something that we could not imagine. Spectroscopy and spectrometers have a fascinating future coming.
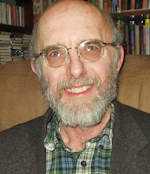
Jeff Hecht | Contributing Editor
Jeff Hecht is a regular contributing editor to Laser Focus World and has been covering the laser industry for 35 years. A prolific book author, Jeff's published works include “Understanding Fiber Optics,” “Understanding Lasers,” “The Laser Guidebook,” and “Beam Weapons: The Next Arms Race.” He also has written books on the histories of lasers and fiber optics, including “City of Light: The Story of Fiber Optics,” and “Beam: The Race to Make the Laser.” Find out more at jeffhecht.com.