Photonics Applied: Standoff Spectroscopy: Standoff LIBS for explosives detection - challenges and status
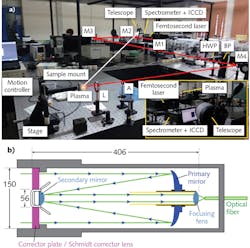
SHAIK ABDUL KALAM, EPURU NAGESWARA RAO, and SOMA VENUGOPAL RAO
Detection of explosives (particularly in the standoff mode) is a significant challenge that has engaged numerous research teams in the last decade.1 Even though much progress has been made in laser-based spectroscopy, effective standoff detection faces two main challenges. The first is sensitivity—the capacity to detect the response from (trace) molecules located a few meters away, while the second is specificity—the ability to provide distinguishable responses (particularly for the different molecules contained in different explosives and for non-explosive materials).
Raman spectroscopy and laser-induced breakdown spectroscopy (LIBS) are probably the only two laser-based analytical techniques that can genuinely address both the sensitivity and specificity requirements.
To date, there has been worthy (but limited) progress in the detection of explosive molecules using the LIBS technique. Compared to nanosecond pulses, femtosecond pulses offer lower continuum generation, lower ablation threshold, and more precise substrate interrogation.2 Furthermore, femtosecond pulses possess the unique property of being deliverable at long distances (meters to kilometers) through the process of plasma filamentation.3
Femtosecond LIBS progress and challenges
Several groups (Laserna and Miziolek) have demonstrated LIBS explosives detection in standoff mode using nanosecond laser pulses for explosive residues on a solid surface, and have even demonstrated detection of residues from behind a physical barrier.4,5 Moros and colleagues accomplished standoff detection using an integrated, mobile platform based on both LIBS and Raman techniques.6 And Gaona et al. provided new insights into the potential factors affecting the LIBS spectra in a standoff configuration, and further demonstrated a supervised learning method for explosive fingerprint identification on solid surfaces.7 Despite this progress, key challenges remain.
When delivering either nanosecond or femtosecond pulses to the point of interest in the field, environmental effects play a crucial role in degrading the laser beam profile or changing the peak intensity, thereby affecting plasma formation. The LIBS system designer needs to apprehend whether a single lens is sufficient for delivering the laser pulses for different standoff distances.
In terms of eye safety, the LIBS technique uses high-energy laser pulses for creating the plasma. Visible laser pulses are not safe for human eyes and this is of great concern when implementing LIBS in places with high human concentration. For the case of landmines, however, this may not be of great concern.
The primary challenge for standoff LIBS is achieving adequate signal detection from the plasma. Effective plasma creation from the laser, the coupling of light to the detector (free-space or fiber-coupled), and the detector sensitivity are crucial issues in achieving superior signal-to-noise ratio. For recording a weak LIBS signal, system designers often turn to a complicated/advanced intensified charge-coupled-device (ICCD) with gating option. Signal optimization and reducing the background/continuum noise in each case are also important.
When using nanosecond pulses, there is a strong chance that the LIBS signal encompasses erroneous contributions from the substrate onto which the explosive residue has been placed. However, ultrashort laser pulses can significantly reduce this possibility during ablation since the interaction time is much shorter and the interaction volume is much smaller.
Improving femtosecond LIBS
Using femtosecond pulses, a Schmidt-Cassegrain telescope (SCT) to collect the light, and a focusing lens to couple it to an optical fiber bundle that transmits the plasma emission to a spectrometer, the ACRHEM Centre at the University of Hyderabad has developed a standoff LIBS system to experimentally determine the limits of the instrument for standoff detection (see Fig. 1).
In the SCT, the incoming light passes through the Schmidt corrector lens (also called a corrector plate) at the front of telescope. It is reflected from a concave primary mirror at the back of the scope that focuses the light to the front of the telescope where it is reflected again by a smaller, convex secondary mirror. Finally, the light travels back through a hole in the primary mirror to the rear of the SCT. Further improvements in the standoff design are possible, as has been demonstrated by Barnett et al., wherein a spatial heterodyne spectrometer is used along with a small telescope to increase the collection efficiency.8
Standoff LIBS measurements at 8.5 m using 50 fs pulses and a 100 mm convex focusing lens for our system can easily show most of the significant spectral peaks of common metals, including stainless steel, brass, copper, and aluminum (see Fig. 2). Furthermore, the instrument displays the spectra of novel explosive molecules of 3-NPY (3-nitropyrazole, C3H3N3O2), 4-NPY (4-nitropyrazole, C3H3N3O2), 1,3-DNPY (1,3-dinitropyrazole, C3H2N4O4), and 1M-3,4,5-TNPY (1 methyl, 3,4,5-trinitropyrazole, C4H3N5O6)-molecules with complex combinations and ratios of carbon (C), nitrogen (N), oxygen (O), and hydrogen (H) elements.Because the CN and C2 peaks are evident from the spectra, these data results, coupled with a few validation models, should provide clear discrimination of explosives and other molecules. In all cases, the samples were translated so as to interrogate a fresh area for different pulses.
In a further experiment, a 3D plot of the combinations of CN/H, C/O, and H/O ratios was obtained from short-distance LIBS measurements of five different bulk explosive molecules (ANTA, DADNE, HMX, NTO, and RDX; see Fig. 3). The points corresponding to each sample were distributed in the 3D volumetric space and the CN-388.2 nm, H-656.2 nm, O-777.2 nm, and N-868.6 nm peaks were chosen in the present study.There were a total of 190 independent spectra recorded, and areas of the peaks were calculated using a Lorentzian fit and then divided by their transition probability and statistical weight of that transition. While the results are impressive for femtosecond LIBS at these 8.5 m distances, true standoff detection at longer distances faces numerous challenges and requires more study, further experimentation, and improved femtosecond lasers.
Further developments
Filamentation studies, improved detection methods, and deeper understanding of the spectra of chemical mixtures are needed to improve standoff femtosecond LIBS.
Harilal's group has accomplished a good amount of initial work on filamentation studies.9 From a comprehensive comparison of femtosecond plasmas under three different conditions (sharply focused femtosecond pulses, loosely focused beam for filament generation, and freely propagating filaments), it was found that a sharply focused femtosecond laser geometry demonstrated the highest persistence of atomic and molecular emission features, plasma temperatures, and densities when compared to the loose and free geometries.
In addition, Harilal's group performed detailed experiments to understand the dynamics of molecular emission from nanosecond, femtosecond, and filament ablation processes.10 Their main supposition was that the persistence of molecular emission was significantly limited in the case of a filament-assisted ablation process. They also observed a delayed molecular emission from the nanosecond laser-induced plasma. Such findings could be useful in exploring new methods for detecting explosive molecules both in pure form and in mixtures.
Beyond a better understanding of how filamentation improves when focusing short laser pulses with variable focal length lenses, the efficacy of ICCDs also merits further exploration. Because they are bulky, complicated, and expensive, recent work in replacing ICCDs with handheld spectrometers is promising.11
Finally, the most challenging aspect of explosives detection is when these explosives are in mixed form (combined with soil or other substances). Very few studies have addressed this issue, but it is imperative to completely understand the role of both the substrate and the mixture.
Towards the ultimate implementation of femtosecond LIBS in standoff mode, the laser group at ACRHEM has demonstrated higher probability of molecular formation in femtosecond LIBS spectra compared to nanosecond LIBS spectra for common explosive molecules such as RDX, TNT, and NTO; that molecular species play a significant role in identification of explosives using LIBS; obtained correlation between the molecular structure and femtosecond LIBS data for a series of explosive molecules; and implemented multivariate chemometric analysis for classification using nanosecond LIBS data.12-14
Myakalwar et al. have unambiguously established that even a small part of the LIBS spectra (and not the entire spectra) is sufficient for explosives detection, thereby reducing the computational analysis time when a large number of spectra are utilized.15 This same group has also shown that non-gated detection is a powerful tool for classification in the case of pharmaceuticals, and has established the effectiveness of LIBS techniques for fingerprinting of structural isomers—an important aspect of explosives detection.16
The holy grail of worldwide standoff explosives detection efforts is to develop a portable system that unfailingly collects LIBS data for various standoff distances, can scan the target and operate in single pulse mode to obtain signals from trace samples in a short period of time, and provide the least amount of false alarms. Hybrid techniques such as LIBS-Raman or nanosecond-femtosecond LIBS could provide additional data-handling support and improved detection. But for femtosecond LIBS to meet niche applications for standoff explosives detection, more research is required.
ACKNOWLEDGEMENTS
We would like to thank the Defence Research Development Organization (DRDO) of India for continued financial support. We also acknowledge Dr. S. Sreedhar for some of the femtosecond LIBS data, as well as the technical support of Krishna Kumar and his team at LightMotif (Hyderabad, India) for designing and delivering the standoff optics.
REFERENCES
1. P. M. Pellegrino, E. L. Holthoff, and M. E. Farrell, Laser-based Optical Detection of Explosives, CRC Press (2015).
2. T. A. Labutin et al., J. Anal. At. Spectrom., 31, 1, 90-118 (2016).
3. A. Couairon and A. Mysyrowicz, Phys. Rep., 441, 2-4, 47-189 (2007).
4. J. L. Gottfried et al., Anal. Bioanal. Chem., 395, 283-300 (2009).
5. R. González et al., J. Anal. At. Spectrom., 24, 8, 1123-1126 (2009).
6. J. Moros et al., Anal. Chem., 82, 4, 1389-1400 (2010).
7. I. Gaona et al., J. Anal. At. Spectrom., 28, 1750-1759 (2013).
8. P. D. Barnett et al., Appl. Spectrosc., in press; doi:10.1177/0003702816687569 (2017).
9. S. S. Harilal et al., Opt. Express, 24, 17941-17949 (2016).
10. S. S. Harilal et al., J. Anal. At. Spectrom., 31, 6, 1192-1197 (2016).
11. A. K. Myakalwar et al., PLoS ONE, 9, 8, article e103546 (2014).
12. S. Sreedhar et al., Spectrochim. Acta B, 87, 121-129 (2013).
13. E. Nageswara Rao et al., J. Anal. Atom. Spectros., 31, 737-750 (2016).
14. M. Ashwin Kumar et al., Talanta, 87, 53-59 (2011).
15. A. K. Myakalwar et al., Sci. Rep., 5, 13169 (2015).
16. A. K. Myakalwar et al., Analyst, 141, 10, 3077-3083 (2016).
Shaik Abdul Kalam is a PhD student, Epuru Nageswara Rao is research associate, and Soma Venugopal Rao is professor, all in the Advanced Centre of Research in High Energy Materials (ACRHEM) at the University of Hyderabad, Hyderabad, India; e-mail: [email protected]; http://www.acrhem.org/venu_home.html.