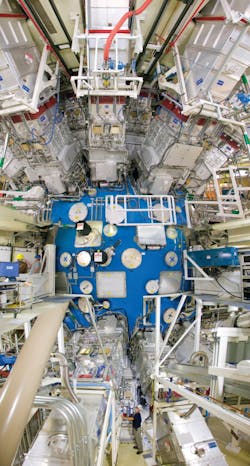
GARY SIMS and DANIEL A. GILMORE
Modern CMOS image-sensor technology has provided a path for routine imaging in challenging environments, including consumer electronics, automotive, and industrial machine vision. The CMOS image sensor’s high degree of electronic integration, low power, and small size have made possible these applications, where temperature extremes, vibration, and shock are regularly encountered.
This article highlights imaging in even more extreme environments, where innovative implementations of a CMOS image sensor in a system have enabled digital imaging over a wide spectral range in environments where it was previously impractical.
CMOS image sensors are routinely used in the high vacuum and radiation environment of space, but the imaging systems employed on spacecraft are typically highly specialized and extremely expensive. Mechanical and optical monitoring and measurement needs in terrestrial harsh environments, including those of high radiation, high vacuum, severe electromagnetic pulse (EMP), and strong shock and vibration, are needed at a reasonable implementation cost.
Application requirements: the challenges
High-altitude astronomical observatories constitute one example of high-performance digital imaging applications in terrestrial extreme environments. For example, the 2.5 m infrared (IR) optical Kunlun Dark Universe Survey Telescope (KDUST) is planned for installation at the Chinese Antarctic Kunlun Station, located in Antarctica’s Dome A ice plateau at 4087 m elevation where ambient temperatures reach -80°C or less.
Other extreme terrestrial environments include those in scientific laboratory applications such as high-energy physics and testing for space-based systems. Additional examples include monitoring processes in vacuum chambers used in semiconductor thin-film deposition or for nuclear-fusion experiments; electron microscopy; and survey and inspection of ocean bottom structures and submerged equipment in nuclear-fission power plants.
Equipment used in research laboratories such as Lawrence Livermore National Laboratory (LLNL)’s National Ignition Facility (NIF) must cope with vacuum issues associated with the target chamber. The cameras are fielded inside the NIF target chamber and therefore need to meet the NIF target chamber cleanliness requirements (see Fig. 1). These are more stringent than typical vacuum cleanliness requirements to protect sensitive optical surfaces. Radiation challenges at NIF are in the form of extremely short, intense radiation bursts.
In test chambers with radiation or vacuum exposure, cable feedthroughs are required to the extreme environment at a cost that can be even more than that of commercial off-the-shelf (COTS) CMOS machine vision cameras. This quickly makes an apparently affordable camera unaffordable. Since few off-the-shelf solutions exist, feedthroughs are commonly custom-built for the specific application.
For high-performance digital imaging systems operating underwater, a limited wavelength range is typically used for optimum transmission through the water while minimizing backscatter for best image quality. Optimizing the sensor for maximum sensitivity at the wavelength of illumination helps to achieve the best image quality possible.
CMOS scientific cameras in use in extreme environments
Earlier this decade, neutron yields at the NIF increased to the point that radiation damage to the sensor, and failure of the supporting electronic components, made the use of CCD sensors untenable. Whereas both CCD and CMOS sensors suffer increased dark current and noise from exposure to radiation, CCD sensors suffer far more due to the additional factor of loss of charge-transport efficiency (a CCD must transport charge across the entire sensor during readout, while a CMOS sensor only transfers charge within a pixel).
In response to the need for more radiation-tolerant imaging systems, Spectral Instruments (Tucson, AZ) developed the 1600S CMOS camera system for use in streak and framing cameras and pinhole x-ray cameras. These camera systems use cooled sensors and are capable of precision, radiometrically accurate measurements. During this development, another need was recognized for a simple machine-vision-like camera that could be used for less demanding applications, yet still survive the intense pulse of 14 MeV neutrons plus associated gamma and x-ray radiation. COTS machine vision cameras were being used for these applications, but were destroyed at a rate of approximately 10% loss for each high-yield experiment.
From this need, the Spectral Instruments RVT100 was developed, using similar architecture and selected radiation-tolerant components as the 1600S, but without the sensor cooling requirements and using a much more cost-effective sensor. Care was taken in the design to provide for conductive cooling of electronic components to the camera case, careful selection of materials in the camera head, and a protocol of cleaning the camera head components to make possible the use of the camera head in the NIF target chamber’s high vacuum without harmful outgassing. The camera withstands 1 × 1016 neutrons at a distance of 5 m—amounting to on the order of 1 × 109 n/cm2—or about 1 rad total dose and continues to operate normally after each experimental shot.
The RVT100’s design philosophy places as few electronic components as possible in a camera head that operates in the radiation/vacuum environment. This both minimizes the number of components placed in a damaging environment (radiation) and minimizes power dissipation where it’s most difficult to deal with (vacuum). The camera head connects to a separate GigE Vision Camera Server outside the harsh environment via a fiber-optic data connection up to 500 m in length. The Camera Server connects to a computer using Gigabit Ethernet for control and data. A recently added feature is a dynamically adjustable power supply for the camera head that maintains adequate voltage headroom for operation, while minimizing heat dissipation—especially important in vacuum environments.
The RVT100 uses a COTS image sensor with a 5.5 μm pixel size and a 1088 × 2048 or 2048 × 2048 image format. It is available as either color or monochrome; the monochrome version can be modified to remove the microlenses to make the part sensitive throughout the UV and soft x-ray region up to 10 keV energy. The combination of a flexible image sensor in a camera system that is radiation-tolerant, EMP-resistant, and operable in a high vacuum gives researchers a new tool for solving challenging imaging problems.Applications using the RVT100
At the satellite test facility at the National Aeronautics and Space Administration (NASA) Goddard Space Flight Center, for example, scientists did a functional test of the RVT100 in a vacuum chamber at temperatures as low as -143°C (see Figs. 2 and 3). This was in preparation for possible testing with the Thermal Infrared Sensor 2 (TIRS-2), a two-band thermal imaging sensor that’s one of the instruments in the Landsat 9, expected to launch in December 2020.NASA also plans to use the RVT100 to provide optical metrology of its Stimulus of Ray Cones (SORC) system with the Wide Field Instrument (WFI) of the Roman Space Telescope, formally known as the Wide Field Infrared Survey Telescope (WFIRST). SORC’s purpose is to provide a means of optical verification of WFI requirements. The camera will operate in a vacuum chamber and cold (220 K) thermal environment. It will acquire images of targets on various filter elements and a reference alignment tool to relay positional knowledge.
Another extreme-environment application uses the new camera at the world's first hard x-ray free-electron laser, the Linac Coherent Light Source (LCLS), at SLAC National Accelerator Laboratory (Menlo Park, CA). The camera is mounted on a microscope within a 2-m-diameter vacuum chamber, at ~10-5 mbar.
The microscope, with the camera on it, is looking at the interaction point of an optical laser (either a highly intense Ti:sapphire laser, or a highly energetic Nd:glass laser) and the LCLS x-ray laser. The optical laser creates dense plasma or matter under high dynamic pressure, and the x-ray laser is used to investigate with diffraction or imaging. The overlap of the optical beam and the x-ray beam on the sample is critical since the spots can be very small (a few microns). Therefore, the microscope must be close to the sample in the vacuum chamber to ensure the laser spots overlap.The company’s current laser scanners work by projecting a laser line onto the target and capturing reflections in a camera module. The scanner rotates to move the laser line across the target and builds up a complete 3D model with millimeter accuracy.
2G Robotics has performed initial testing on an RVT100, with promising results, to further improve performance and longevity for use in a radiation-hardened laser scanning system currently being developed for nuclear powerplant inspection.
The company plans on further testing of the RVT100 camera to ensure that it operates in the extreme-radiation environment near the reactor core, and at the rates and sensitivity levels required to work as a reliable and robust component in its new inspection system. 2G Robotics has noted that their research found that the RVT100 is the only machine-vision camera available that combines radiation hardening, a GigE interface, OEM-readiness, low power use, and a small-enough form factor for its new nuclear inspection systems’ needs.
The ability of these CMOS-sensor-based systems to handle such demanding applications is only the start of what can be achieved. Sensors with higher resolution, greater spectral range, sensitivity to subatomic particles, and higher frame rate are all possible for applications ranging from the study of nuclear processes to the quality control of vacuum web coatings. In between these extremes are a host of potential applications such as monitoring the position and temperature (using an IR-sensitive sensor) of samples in electron microscopes, monitoring the position of optical, x-ray, and electron beams in synchrotrons, and measuring the velocity and trajectory (using high-speed sensors) of mechanical assemblies in semiconductor wafer handling equipment.
Gary Sims, Ph.D., is cofounder, president, and CTO and Daniel A. Gilmore is application scientist, both at Spectral Instruments, Tucson, AZ; ; e-mails: [email protected] and [email protected]; specinst.com.
FOR MORE INFORMATION
National Ignition Facility (NIF)
NASA Thermal Infrared Sensor 2 (TIRS-2)
Roman Space Telescope
Linac Coherent Light Source (LCLS)
2G Robotics