Optoacoustic Imaging/Photoacoustics: The wide-ranging benefit of photoacoustic commercialization
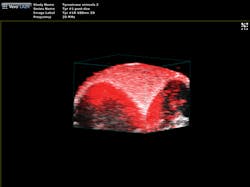
Biomedical imaging has enabled many advances, thus feeding and sustaining a thirst for better ways to visualize what can't be seen with the naked eye. From x-ray and ultrasound to confocal microscopy and optical coherence tomography (OCT), each new modality offers some balance among resolution, scope, and functionality to yield helpful details about what makes our bodies tick.
A duo for depth
Of course every modality has some restictions. Optical techniques are impacted by light scattering, which limits the amount of information that can be captured and the quality of images produced. But photoacoustic imaging (a.k.a. optoacoustic imaging) combines the best of two worlds to overcome this limit, and provides unique benefit to boot. Optical stimulation of acoustic signals enables multi-scale, high-resolution, noninvasive imaging of both structure and function deep into tissue.
"When you are talking about going inside the body, you need infrared light, between 650 and 1000 nm. That will get you a few centimeters' penetration into the human body, which is quite a bit," said Eli Margalith, CEO of Opotek, which supplies Nd:YAG-pumped optical parametric oscillators (OPOs) to research labs and equipment manufacturers for their photoacoustic imaging systems. Thanks to the use of OPOs for acoustic signal creation, many photoacoustic imaging systems allow wavelength tunability, so a single device can serve multiple applications.
"Photoacoustic imaging is showing real promise as an alternative to MRI and CT scans," added Patrick Maine, president of Quantel Laser, which makes the Nd:YAG lasers used in Opotek's OPOs. "We are seeing strong research interest driven by the high resolution enabled by this technique. And it is encouraging to see this beginning to move out of the lab and into clinical applications."
The photoacoustic effect was discovered by Alexander Graham Bell in the 1800s, but it took another century for the technology to catch up.1 In photoacoustic imaging, a short-pulsed laser is used to produce ultrasound in tissue. When the laser energy hits the target tissue, it heats and expands, generating an acoustic signal that can be reconstructed to show the distribution of optical absorption inside the target.
Because the ultrasonic scattering coefficient in tissue is two to three orders of magnitude less than its optical counterpart, photoacoustic imaging overcomes the optical diffusion limit (~1 mm in the skin) found in high-resolution optical-contrast imaging. And because either unscattered or scattered photons can trigger the photoacoustic signals, photoacoustic waves can be generated several centimeters deep into tissue—currently 1–7 cm.2 As a result, light-absorbing structures inside these tissues can be detected and visualized with resolutions far superior to optical methods at those depths and without the need for biomarkers.
Uniquely reveals function
"The functionality you get from photoacoustic imaging is key," Margalith said. "When you do ultrasound, you get morphology, you get information about different organs and where they are, but you do not have any information about functionality. With the laser as the initiating mechanism for the ultrasound, functionality becomes the main reason. You can measure, for example, the oxygen level in the blood in real time. You cannot do that in any other imaging modality."As a result, the biomedical research community has been excited about the possibilities of photoacoustic imaging for decades. Preclinical applications include the study of non-fluorescent pigments, angiogenesis, microcirculation physiology and pathology, drug response for screening, and brain functions. In addition, photoacoustic microscopy and photoacoustic tomography devices are being developed for a variety of clinical point-of-care applications, including cancer screening, brain imaging, intravascular catheter imaging, chemotherapy monitoring, and blood flow/oxygenation imaging (see Figs. 1-3).
For example, Lihong Wang, a professor of biomedical engineering at Washington University in St. Louis (Missouri) and a pioneer in the development of photoacoustic imaging techniques and technologies, has made major inroads in the study of the brain. His research has attracted nearly $50 million in grant funding, including a three-year, $2.7 million award as part of the BRAIN Initiative, and his lab (now relocating to Caltech in Pasadena, CA) is credited with being the first to report on functional photoacoustic tomography, 3D photoacoustic microscopy, and photoacoustic endoscopy, among many others. In spring 2015, they announced yet another photoacoustic breakthrough: the ability to visualize single capillaries and blood oxygenation in the brain through the intact skull of a mouse.Market emergence
Slow to start, commercialization of photoacoustic imaging is at last gaining momentum. TomoWave (Houston, TX) a startup founded by optoacoustics pioneer Alexander Oraevsky, is commercializing the Laser Optoacoustic Ultrasonic Imaging System Assembly (LOUISA) for breast imaging. The system provides 3D full-view optoacoustic tomography that works in conjunction with laser-generated ultrasound to produce functional and anatomical maps with high sensitivity, high resolution, and minimal artifacting or distortion.3 And Seno Medical Instruments, which acquired the rights for the first core patent by Oraevsky, is leading the commercialization of a handheld two-dimensional optoacoustic and ultrasound device based on that patent.
Meanwhile, the European FULLPHASE project launched in October 2012 is making headway in its development of a portable, multiwavelength photoacoustic/ultrasound system for point-of-care disease detection and treatment monitoring in oncology, rheumatology, and cardiovascular disease (see Fig. 4). Among the project participants is Quantel, which was selected to provide the diode lasers for this program because of the company's unique ability to efficiently package high-power quasi-continuous-wave (QCW) diode stacks and arrays, according to Andreas Kohl, technical lead for Quantel's diode laser group."The diodes we provide are very short pulse, about 90 ns or less, so for penetration depth we also need high pulse energy," Kohl said. "We started with 1 mJ and are now going up to 4 mJ. And that is where it gets critical, because if you have high pulse energy and short pulses, you have very high electrical currents driving the diodes, and this is meant to be a handheld device. So you want to make sure the lasers are very efficient because you want to make sure that the generated heat does not burn the hand of the person using it."
Important endorsements
Another sign that photoacoustic imaging is "arriving" in medicine is that large companies are beginning to invest in it. For instance, Canon is commercializing a photoacoustic mammography system developed by the startup OptoSonics (Oriental, NC). And in 2010, Sonosite—a subsidiary of Fujifilm and a leading manufacturer of point-of-care ultrasound equipment—spent $71 million to acquire VisualSonics, a manufacturer of real-time, ultra-high-frequency micro-ultrasound technology. Now, VisualSonics is marketing the Vevo LAZR photoacoustic imaging system that offers a selection of modalities, from micro-ultrasound, Doppler, and microbubble contrast to photoacoustic. The system uses an OPO pumped by a doubled Nd:YAG laser that is tunable from 680 to 970 nm, and features a repetition rate of 20 Hz, pulse width of 5 ns, and pulse energy of 50 mJ.
"We are a preclinical ultrasound company that is using pulsed laser light to generate acoustic signals," said Drew Heinmiller, photoacoustics product manager at VisualSonics. "The OPO allows us to change the wavelength of the laser, which is key to being able to do just about anything in photoacoustic imaging."
In addition to its conventional cardiovascular market, VisualSonics is targeting applications in cancer research, where the ability to tune the laser to measure blood oxygenation levels in real time is a major advantage for tumor monitoring and analysis.
"We can tune the laser and do a calculation to show in an image, with very high resolution, what the oxygen saturation status of the blood is," Heinmiller said. "So now we measure how much oxygen the blood is carrying in real time, in vivo, in animal models."
Combining photoacoustic imaging with ultrasound offers additional insights into the disease process, he noted.
"One of the benefits of having ultrasound in this system is that you can use it to find the tumor, and then you can overlay the oxygen information on top of the ultrasound image and it gives you a physiologically relevant parameter (oxygen saturation) and allows you to place the tumor within the anatomy," Heinmiller said. "If you're using just photoacoustic imaging, you would be looking at blood vessels, vasculature, etc., but you wouldn't necessarily know exactly where it was located."
Also entering the commercial sector is another photoacoustic imaging pioneer, John Viator, who began his biomedical research career in 1995 in Steve Jacques' lab at Oregon Health and Science University (Portland, OR). Viator's company, Acousys Biodevices, is focused on using photoacoustic flow cytometry to detect circulating tumor cells, a technique he invented while at the University of Missouri. His company is initially targeting melanoma, the most aggressive type of skin cancer.
"We use the Opotek device in our laboratory since it is an outstanding tunable source," Viator said. "While the OPO was critical in our laser wavelength studies in device development and engineering, our commercialized device will probably be a single wavelength device, since it doesn't need the flexibility of the OPO."
Acousys' device emits laser light into enriched blood cell samples to determine whether cancer cells are lurking among millions of white blood cells. Melanin within the cancer cells uniquely absorbs the light, which generates high frequency acoustic responses detected by sensors in a photoacoustic flow meter. The device has been shown to detect and capture as little as a single melanoma cell from a sample of 10 mL of blood, and sample processing takes only 20–30 min. Captured cells can be further analyzed.
"Once you capture these individual cancer cells, you can do molecular tests, genetic tests, image them under a microscope, and learn more about that particular cancer and how it's spreading," said Viator, who, in addition to heading Acousys, leads the biomedical engineering program at Duquesne University. "Instead of blindly prescribing chemotherapies, if you capture the individual cells that are spreading, you can verify the type of melanoma that responds well to a certain drug."
It also offers a number of advantages over other emerging methods for this application, he noted.
"As far as finding circulating tumor cells or other pathological particles in body fluids, photoacoustics, number one, is gentle," Viator said. "You're just sending some photons to it, not chemically altering it or destroying the cell. You're not making contact through some sort of substrate, just keeping it in the body fluid and sensing it by adding a little laser energy and then listening to the acoustic response. And it is fairly rapid compared to competing technologies for finding pathological particles in body fluids."
Opportunity for photonics
These advances bode well for component manufacturers. Gregory Smolka, VP of sales and marketing for Quantel, describes a long history of creating OEM sources for biomedical devices, and years of effort in photoacoustics, working with groups using pulsed Nd:YAG directly, as well as those using different wavelengths via OPOs or dye lasers. "We're investing to help develop this rapidly emerging application," Smolka says—an application that portends great benefit for photonics developers, systems innovators, and for patients.
REFERENCES
1. P. Shao, IEEE J. Transl. Eng. Health Med. (Mar. 19, 2014); see http://bit.ly/1SrXxez.
2. L. Wang, Scholarpedia, 9, 2, 10278 (2014).
3. L. Mertz, IEEE Pulse, 6, 3, 28–33 (May/June 2015); doi:10.1109/mpul.2015.2409099.
About the Author
Kathy Kincade
Contributing Editor
Kathy Kincade is the founding editor of BioOptics World and a veteran reporter on optical technologies for biomedicine. She also served as the editor-in-chief of DrBicuspid.com, a web portal for dental professionals.