SPECTRAL IMAGING: Spectral imaging provides new view for biotech and medical professionals
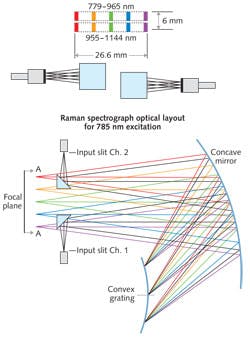
CHRISTOPHER VAN VEEN
Spectroscopic instruments have often suffered from being overly complex and costly; as a result it has taken awhile for spectral sensors to become more mainstream and easier to use within the healthcare and biomedical markets. But these fields are where spectral sensing—in all its forms—is paying tremendous benefits with respect to improving healthcare diagnostics and enhancing the quality of life.
In medical applications, there are two spectroscopic methods of general interest: Raman imaging and hyperspectral imaging. These methods can be used for noninvasive healthcare screenings, genomics, and pharmaceutical production.
In each case, the objective is to "see" what cannot be seen by the naked eye, by looking at the spectral signatures within the sample. The advantage of spectral imaging over single-point laboratory instruments is that larger objects (such as an assay array, tissue sample, or a human hand) are spectrally and spatially mapped at high resolution. Coupled with spectral libraries representing hundreds of individual signatures, pathogens or biomarkers can be measured, mapped, and reported in real time. With advances in data processing and bio-informatic analysis, spectral systems perform painstaking work more quickly and cheaply, and can be deployed in diverse medical settings.
Raman spectroscopy for the biomedical arena
Raman spectroscopy is a laser-based technique for the ultraviolet (UV), visible (VIS), and near-infrared (NIR) regions. Samples are excited using a laser that induces a reliable Stokes "fingerprint" that allows for accurate sample identification. Common excitation wavelengths include 248, 355, 532, 685, 632.8, 785, 830, and 1064 nm. The instrument choice depends on the vibrational state of the chemical bonds and the molecular symmetry.
When laser light is absorbed by the sample, the energy vibrates the molecular bonds until they relax and emit a photon of a longer wavelength. Light collected from the sample is first filtered to remove the excitation wavelength and then dispersed into a detector. The measured spectral response is the unique spectral fingerprint by which the molecule can be identified. By scanning a sample under an excitation laser and a 1D fiber array, a complete Raman spectrum is acquired, producing separate images of the sample at every wavelength in the spectrum. It poses an advantage over traditional single-point Raman systems, because acquiring one spectrum takes milliseconds and a full 3D image takes only a few minutes.
High-resolution Raman spectra make this the preferred technique for biomedical diagnostics and research. However, Raman signals are often very weak. Imaging detectors have only recently matured enough to put high-quantum-efficiency (QE) cameras onto the market at acceptable costs for widespread deployment. With advances in detector technology, indium gallium arsenide (and silicon chips can reach across wider bands with smaller pixels and detect weaker signals.
Similar advances in laser diodes and diode-pumped solid-state lasers allow for less costly, brighter illumination. Although fiber has traditionally been the means of delivery and collection of Raman signals, microscope-based Raman systems are gaining acceptance for many biological samples.
An interesting case study in Raman system deployment is at the Children's Hospital of Orange County (Orange, CA), where the Raman Explorer from Headwall Photonics is being used to identify tumors (see Fig. 1).
Analysis by the researchers showed that the key metric for any Raman system is optical throughput efficiency of the Raman spectra. The instrument improved test speed while maintaining high SNR and resolution across the spectral band. Compared with other instruments, the researchers found that throughput efficiency was up to 221% greater.
The aberration-correction optics also eliminated keystone and smile image degradation. Minimizing these optical aberrations eliminates costly post-processing, rendering distortion-free results faster.
Multipoint spectrometers
In the laboratory, Raman imaging systems make an array of measurements in parallel. Using special optical fibers to handle the laser excitation, these systems simultaneously take Raman spectra from as many as 40 fibers without scanning. While fiber-array instruments often suffer from crosstalk between channels, imagers employing aberration-corrected optics minimize this crosstalk. This allows scientists to obtain accurate detection and identification of single molecules in free-solution environments with high resolution.
Using a fiber array to track molecules in real time down the length of a microfluidic channel enables lab-on-a-chip analysis before, during, and after reaction, continuously, with a single instrument.
Raman imaging opens up opportunities for time-resolved investigative analyses into biomarkers, DNA fragments, polymorph screening, and genomics. In the past, this amount of data may have been unwieldy, even in a laboratory setting. But data-processing power continues to advance according to Moore's Law, making simultaneous, time-resolved measurements more affordable.
As a result, university laboratories and medical research institutions are able to invest in the technology and refine it for deployment in the clinic. For example, an instrument developed by the Massachusetts Institute of Technology (MIT; Cambridge, MA) provides a noninvasive way of monitoring blood glucose levels. After scanning a patient's arm or finger with NIR light, the instrument reads the glucose level directly from the collected Raman spectrum and eliminates the need to draw blood. The size of the instrument—about that of a standard notebook computer—means that it can be used in a doctor's office or as a home kit.
Hyperspectral imaging
Hyperspectral imaging is another major category for deployment of spectral sensors for reflective spectroscopy. The pharmaceutical industry is one area in which hyperspectral imaging is making an impact.
The United States Food and Drug Administration (FDA) seeks to achieve quality uniformity across the industry; process analytical technology (PAT) is the term used to describe this effort. PAT involves defining the critical process parameters (CPPs) of the equipment used to make and inspect the product, which affect the critical quality attributes (CQAs) of the product. Hyperspectral imaging can help control CPPs within defined limits. Because hyperspectral systems can see with high spectral resolution, pharmaceutical manufacturers can monitor and inspect their products for quality in real time. Continuous feedback from hyperspectral data helps engineers to better understand how manufacturing parameters change under different conditions, reducing waste and costs.
Additionally, hyperspectral systems can play a role in the formulation of pharmaceuticals by instantly recognizing and categorizing active pharmaceutical ingredients (APIs) and measuring samples for purity (see Fig. 2). Utilizing what are known as spectral libraries, chemical compounds can be analyzed and categorized instantly across an array of common or completely different chemicals. For example, four seemingly identical white tablets representing aspirin, acetaminophen, vitamin C, and vitamin D can pass within the field of view of a hyperspectral instrument. To the system, the spectral fingerprint for each is immediately recognized by the sensor, even though the naked eye sees four identical white tablets. Deploying the same technology in a clinical setting could help medical staff catch a mistakenly dispensed drug before it harms a patient.Developing systems that meet the rigors of the pharmaceutical industry (whether dealing with formulation—the API—or high-speed inspection) means that certain characteristics need to be optimized. Distorted images are unacceptable to manufacturing or research and are eliminated thanks to the use of aberration-corrected optics that deliver a properly proportioned and focused image. The field of view (FOV) for process lines differs from what would be needed for lab samples, and hyperspectral systems have tall slits for a wide FOV.
The instantaneous field of view (IFOV), or spectral/spatial FOV, can have high or low resolution depending on the application, or asymmetric resolution if only spatial resolution needs to be high. Stacking thousands of these IFOV snapshots like a deck of cards yields a hyperspectral data cube containing two spatial directions and one spectral direction. This data cube contains a wealth of information that is managed and analyzed in real time with the help of a data-processing unit. Constantly advancing processor and storage technology make hyperspectral analysis speedier and more applicable.
In the same way that NIR hyperspectral imaging has gained interest in the pharmaceutical industry to meet strict process requirements from the FDA, the food-processing industry is also adopting hyperspectral technology to improve food safety and quality. Another instrument, the Hyperspec Inspector, is now deployed across many high-speed food-inspection lines as it looks for and rejects contaminants having the wrong spectral fingerprint (see Fig. 3 inset).Companies such as Headwall have teamed with United States Department of Agriculture (USDA) researchers to advance in-line inspection technologies for a wide range of food groups, including poultry and specialty crops, among others. The USDA is also showing strong interest in hyperspectral analysis because the agency believes that food quality and security will be improved through the use of these systems across the processing industry. Processing companies see the benefit, too, because they can demonstrate a quantifiable return on the investment: Waste is minimized, speed is maximized, and factory throughput is optimized.
Nanobead indicators
Another area of tremendous promise for hyperspectral imaging is the microscopic analysis of nanobead indicators. For applications such as tracking and classification of cellular drug absorption and delivery, or quantifying the presence of tagged nanobeads within tissue samples, hyperspectral imaging represents a valuable extension of traditional research techniques that can utilize existing optical microscopes available within the laboratory. With research samples positioned along the microscope stage, spectral imaging yields critical analytical information with the addition of a hyperspectral sensor attached with a C-mount adapter to the exit port of the microscope.
Christopher Van Veen is marketing manager at Headwall Photonics, 601 River St., Fitchburg, MA 01420; e-mail: [email protected]; www.headwallphotonics.com.