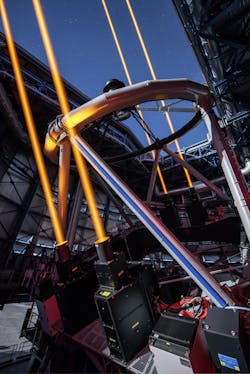
MARTIN ENDERLEIN, WILHELM G. KAENDERS, and DOMENICO BONACCINI CALIA
The chance to let powerful visible lasers escape into the open sky is a dream for every laser engineer—yet having four of them at the same time 'imprinting' impressive columns of light into the clean air of the desert and aiming at the Southern Cross constellation was the visual highlight of a recent event (see Fig. 1).On April 26, 2016, the southern skies witnessed the "first light" of four new 20 W-class sodium guide star lasers pointing to the stars at the European Southern Observatory's (ESO; Garching, Germany) Paranal Observatory in the Chilean Atacama desert.1 With this event, a seven-year contract and collaboration between laser suppliers Toptica Photonics (Graefelfing, Germany) and MPB Communications (MPBC; Montreal, QC, Canada), and ESO as development partner and customer, comes to a successful finish.
This first light event also marks an important milestone in a major upgrade of ESO's Very Large Telescope (VLT) to transform the Unit Telescope 4 into a state-of-the-art adaptive telescope facility (AOF).8 The transformation will involve further steps: in late 2016 with the installation of a new 1.1 m deformable secondary mirror, and in 2017 with the commissioning of the two adaptive optics modules: GRAAL and GALACSI.
Adaptive optics systems consisting of natural and/or artificial guide stars, wavefront sensors, real-time computers, and deformable mirrors are the method of choice to counteract the image-blurring effects of unavoidable atmospheric turbulence at ground-based optical telescopes. In fact, even at the best optical sites on Earth such as the 2600 m summit of Cerro Paranal or the 4205 m summit of Mauna Kea in Hawaii, refractive-index variations in the atmosphere lead to a seeing-limited resolution of 0.4 arcsec in contrast to the theoretical diffraction-limited resolution of less than 0.02 arcsec for the 8.2 m VLT telescopes.
Since there are not enough bright, natural guide stars in many parts of the night sky, large telescopes with the small fields of view of an adaptive optics system need to create their own artificial stars. In the 1980s, Foy and Labeyrie, and before them Happer and McDonald in a classified JASON report, devised a method to make use of the high concentration of atomic sodium in an approximately 10-km-thick layer that occurs at the edge of the mesosphere, 80–100 km above Earth's surface.2,3 Because of a large product of resonance fluorescence cross-section and abundance (column density of about 4 billion sodium atoms per square centimeter, or 400,000 atoms per cubic centimeter), excitation of these atoms promises high photon return from a location far above all atmospheric turbulences, a favorable condition for full sampling of all the turbulent layers that typically extend to an altitude of 25 km.
A guiding star
The new lasers now installed at the VLT constitute the third generation of sodium guide star lasers, bringing quantum optics technology to the astronomy community.4 Only within the last 10 years, extensive computer simulations have identified the detrimental effects of Larmor precession, off-resonant excitation, and saturation in the atmospheric sodium that has led to the development of this optimized tunable diode laser-based technology. Core components are a stable quantum-dot distributed feedback (DFB) diode as the seed laser, a polarization-maintaining narrowband Raman fiber amplifier building on patented ESO technology and provided by MPBC, and efficient frequency doubling by resonant second-harmonic generation (see image at top of this page).
With more than 80% doubling efficiency, each laser delivers 22 W of diffraction-limited (20 nm root-mean-squared [RMS] wavefront variation), narrowband, continuous-wave (CW) radiation at a sodium resonance near 589 nm, including up to 12% of the power at a second repumper frequency.
Using a second repumper laser at the sodium D2b wavelength enhances the benefits of optical pumping and reduces the depletion of the accessible ground state of the atom—a well-known technology in atomic physics and quantum optics, in particular for laser cooling of atoms. In such experiments on the single atom level, signals are very small and measurements have to be repeated many times for collecting statistics. Therefore, it is essential to reliably reproduce the same procedure despite imperfections in the experimental conditions that can lead to unwanted transitions into (dark) atomic states from which no excitation with the detection laser is possible. The additional repump laser recovers these atoms by inducing transitions from the dark state back into the detectable state.
In adaptive optics, the number of atoms in the sodium layer in the upper mesosphere interacting with the guide star laser is relatively large, as sodium is being continuously replenished by meteors. On the other hand, the "experimental" conditions are more unstable than in a quantum optics lab because of varying geomagnetic field conditions, high altitude winds, and temperatures, leading to Doppler broadening of the atomic lines because of the motion of the atoms. However, recent simulations have clearly shown that a repumper is able to increase the guide star effective brightness—the fluorescence photon return—by a factor of up to four.5
For the first time, this new generation of guide star lasers features an "embedded" repumper. The second laser frequency is realized within the same laser architecture by a frequency modulation of the oscillator. As this can be done electrically by modulating the current of the diode seed laser, any additional components such as electro-optic modulators can be avoided that would additionally disturb the optical wavefront—a very critical parameter for this application.
Using this technique, the performance of this laser system in terms of beam quality and achievable guide star brightness can surpass that of 50 W systems of the previous generation. The artificial stars now reach an apparent magnitude of 6—just at the limit of what the naked eye can see. Typical absolute numbers for return flux are on the order of a few 10 million photons per second per square meter.Quadrupling the impact
Using several lasers, the complete air volume above the telescope's main mirror can be probed in a process called atmospheric tomography. For the first time at the VLT, four CW, 589 nm, 22 W lasers are used in parallel to implement advanced modes of adaptive optics (see Figs. 2 and 3). In this way, apart from near-diffraction-limited images of the complete field of view, better angular resolution can be obtained for smaller fields of interest. The VLT adaptive optics facility will be an important precursor and pathfinder for the 39 m European Extremely Large Telescope (E-ELT) to be built on neighboring Cerro Armazones by 2024.The Four-Laser Guide Star Facility (4LGSF), as part of the AOF,8 enables routine observation of extragalactic objects with laser guide star adaptive optics, as well as observations within our own Milky Way galaxy of areas with fainter stars or star-formation regions obscured by dust.
One object to be explored with the new system will be the alleged gas cloud G2 that in 2014 made its closest approach to our galactic center and its supermassive black hole Sagittarius A-star (Sg A*), whose mass is estimated at 4.3 x 106 solar masses. As it came close to within 20 light hours, astronomers expected to witness the accretion of large parts of the cloud by the black hole. However, recent observations suggest that the cloud survived the encounter relatively undisturbed, fueling new speculations as to the composition of the cloud. This year and the next will be crucial for tracking the cloud's motion after the encounter, and momentum is building to start bringing online the newest detectors with even more resolving power.
Guide star lasers are also being used to help detect space debris. These leftovers from rockets and satellites have become a pressing problem over the last few years, particularly because of a few major incidences such as China's anti-satellite missile test targeting its own Fengyun-1C satellite in 2007 and the collision of satellites Iridium 33 (U.S.) and Cosmos 2251 (Russia) in 2009. Currently, experts cannot rule out that the so-called Kessler's syndrome—a predicted self-enhancing growth in the amount of small debris by accidental collisions—has already been set in motion.
Today, the detection of space debris is mainly accomplished with high-resolution radar technology such as the U.S. Air Force's new Space Fence system that is supposed to see full operation in 2019. The resolution of radars is limited, however. Although there does exist a catalog of roughly 20,000 objects larger than 5 cm in diameter, much smaller particles can also be fatal to satellites and spacecraft since relative velocities in the tens of kilometers per second are not uncommon. There are an estimated 670,000 objects with sizes between 1 and 10 cm, and millions more smaller objects.
Laser guide stars can help detect and track small debris objects in adaptive-optics-assisted optical observations. Apart from that, though, there have been proposals for laser-assisted de-orbiting of space debris.6 The most realistic version (avoiding politically volatile space-based lasers) combines ground-based, high-power infrared (IR) lasers with laser guide star adaptive optics. The laser guide star would have to be robust enough to be moved across the sky synchronous with the debris object, and bright enough for a fast adaptive optics loop to correct the uplink high-power laser beam. Photon pressure or recoil because of laser ablation would slow down the debris object, resulting in faster re-entry and atmospheric burn-up.
When first proposed in a NASA study called project ORION in 1996, it was considered very costly because of the high-power lasers and the large fast-slewing telescope that were required.7 Now is the time to reconsider. High-power laser technology has advanced considerably and robust sodium lasers for fast-slewing telescopes are now available.
Commercial success
For Toptica and MPBC, the collaboration with ESO has—apart from being a lot of fun for the laser experts—triggered much development, both in technology as well as project management and documentation processes. MPBC, who is producing their all-fiber pumps and, under license from ESO, the narrowband Raman fiber amplifiers, was able to introduce an offshoot product line of single-frequency amplification products at many visible and near-IR wavelengths.
In addition, Toptica is offering fully digital second- and fourth-harmonic generation laser systems supporting new applications for the scientific and commercial research community, including remote control and self-optimizing hardware and software features for maintenance-free performance of both astronomical and industrial laser systems.
The appreciation of the wider astronomy instrumentation community for our work is apparent in that all next-generation ELT projects have chosen the SodiumStar as their baseline approach. For the first time, a turnkey, maintenance-free guide star laser system is routinely available as a commercial off-the-shelf product, and recognized as quasi-standard for existing and planned telescopes around the world.
REFERENCES
1. See www.eso.org/public/news/eso1613.
2. R. Foy and A. Labeyrie, Astron. Astrophys., 152, L29–L31 (1985).
3. W. Happer and G. MacDonald, JASON Report No. JSR-82-106, MITRE Corp., McLean, VA (1983), and cited in R. Q. Fugate et al., Nature, 353, 144–146 (1991).
4. See http://bit.ly/1Ygl5oD and B. Ernstberger et al., "Robust remote-pumping sodium laser for advanced LIDAR and guide star applications," Proc. SPIE, 9641, 96410F (Oct. 8, 2015).
5. R. Holzlöhner et al., Astron. Astrophys., 510, A20 (2010).
6. F. Bennet et al., "Adaptive optics to enhance tracking of space debris," Proc. SPIE, 9148, 91481F (2014).
7. J. W. Campbell et al., "Project ORION: Orbital Debris Removal Using Ground-Based Sensors and Lasers," NASA Technical Memorandum, 108522 (1996).
8. R. Arsenault et al., "ESO Adaptive Optics Facility: under test," Proc. AO4ELT3 (2013); http://bit.ly/28YtB6G.
Martin Enderlein is a systems engineer and Wilhelm G. Kaenders is president, both at Toptica Photonics, Graefelfing, Germany; e-mail: [email protected]; www.toptica.com; while Domenico Bonaccini Calia is a physicist at the European Southern Observatory (ESO), Garching, Germany; www.eso.org.