The rapid success of integrated electronics made integrated photonics a logical step. Stewart E. Miller of Bell Telephone Laboratories outlined his plan for “integrated optics” in the September 1969 issue of the Bell System Technical Journal. He envisioned “a miniature form of laser beam circuitry” made by changing the index of refraction of glass substrates by a factor of 1% to 10% to form a 10 μm waveguide. Photolithography—already widely used in silicon—would create complex optical circuits, including miniature lasers, modulators, and other components. According to Miller, his plan “would facilitate isolation of the laser circuit assembly from thermal, mechanical, and acoustic ambient changes through small overall size; economy should ultimately result.”1
He led Bell’s optical communications program at the time, when it still focused on sending laser beams through hollow optical waveguides. It was the kind of long-term effort the old Bell Labs could afford. Fiber optics soon leaped far ahead, leaving integrated optics research to continue in the background.
Sematech and the birth of consortium projects
After more than a quarter-century of leadership in solid-state electronics, the U.S. semiconductor industry was worried about its future in the 1980s. Fearing Japan’s support for its electronics industry endangered U.S. competitiveness, 14 semiconductor companies and the Department of Defense (DoD) in 1987 launched a nonprofit consortium to develop semiconductor manufacturing technology, powered by $500 million of DoD support over five years.
It proved a difficult start-up. In mid-1988, the group lured legendary Intel founder Robert Noyce out of retirement. He passed away in 1990,2 yet Sematech survived and has remained a vital engine of growth for the semiconductor industry. It also would serve as a model for AIM Photonics.
Integrated photonics development
Integrated optics became integrated photonics, and “silicon photonics” became a hot buzzword in the 2000s. However, despite silicon’s value in both optics and electronics, it stubbornly has refused to generate adequate light to sustain integrated photonics.
One alternative has been integrating photonics and electronics in III-V semiconductors such as indium phosphide (InP) that are good light emitters. InP integrated circuits are widely used in high-performance telecommunication systems.3 However, InP electronic circuits are far less mature than silicon electronics, so integrated photonic chips using such materials cannot be scaled to the complexity or manufactured in the quantity and cost as silicon.
The leading alternative is connecting external light sources to the silicon. An optical fiber could deliver light from the external source to a silicon waveguide, or a III-V light source could be flip-chip bonded to the silicon. That combination gives the best of both worlds: high-performance III-V light sources and more complex and powerful silicon electronics.
By a decade ago, integrated photonics had become a chaotic wild west, says Peter O’Brien, head of the photonics packaging group at the Tyndall National Institute at the University of Cork (Cork, Ireland). Every company had their own rules for laying out and packaging and fabricating chips. That gave them the freedom to optimize their design to meet their needs, but it also limited their access to the large-volume manufacturing technology developed by the semiconductor industry.
Sematech’s living legacy has been its development of massive foundries that fabricate millions of transistors and other devices on semiconductor wafers, then slice the wafers into finished chips. The foundries are massive in size and staggering in cost, but they are tremendously efficient at producing very sophisticated chips in very large numbers at modest cost.
Achieving that efficiency requires electronic chip designers to follow stringent rules so they, too, work within the foundry system. Such a system could also work for integrated photonics, but to use it, O’Brien says, “we needed design rules.” The European Photonics Academy has been working to do that, with Tyndall working on the rules. AIM Photonics is leading the effort in the U.S.
AIM Photonics goals
The centerpiece of AIM Photonics is a billion-dollar semiconductor fabrication foundry that does “much more than just photonics,” says Thomas Brown, a professor at the University of Rochester and a leader in developing the group’s test, assembly, and packaging program. Based at the State University of New York Polytechnic Institute (SUNY Poly; Albany, NY) and funded by New York State, the foundry was already being used by SUNY Poly strategic partners on semiconductor electronics when planning began for AIM Photonics. It boasts a full suite of 300 and 200-millimeter chip fabrication.4 Federal agencies and industrial partners also provide funding for AIM Photonics.
“They have the cutting-edge foundry fab tools to do everything needed for photonics, with much more repetition and replicability than in a smaller foundry. And it was built to do 300 mm wafers, which gives you a lot more chips per wafer” and drives down costs (see Figs. 1 and 2), Brown says, noting that developing an optical version of the foundry will come alongside the electronics ones, but not replace them. Optics are superb for long-distance transmission of large volumes of data and are vital for quantum communications, but are much better processing information.
Applications such as data communications that require on-board light sources pose a challenge, and John Bowers’ group at the University of California, Santa Barbara are developing III-V quantum dots and other devices that don’t like to grow on silicon. They would like to find ways to integrate them into the foundry, but it’s tricky to bring in III-V materials without cross-contaminating the silicon. Working out the right process parameters will take time.
“You can’t do anything unless you have design tools,” Brown says. His group has partnered with major electronic and photonic design firms including Cadence (San Jose, CA), Synopsys (Mountain View, CA), and Siemens Design Automation (formerly Mentor Graphics; Plano, TX). Users running the tools can submit their designs to the foundry for production, avoiding the need for their own tiny foundry.
Packaging
Tyndall got into packaging before anyone else was really doing it, and it’s become crucial for integrated optics. It goes beyond the obvious packaging to include electrical and mechanical packaging, thermal issues, and application-specific requirements, including lightweight and minimum size for aerospace equipment, O’Brien says.
AIM Photonics is doing most of its packaging and related work in facilities in Rochester. According to Brown, processing differs from the linear operation of a foundry. A packaging center starts with large wafers, which it divides into individual dies, then places them mechanically on a substrate or carrier, and finally arranged for the application. Details need to be standardized as much as possible, such as maintaining a common distance between fiber arrays or connections. It takes time to work out those standards and accommodate users to them. That can be a particular problem for small companies accustomed to being in full control of their own designs, but standardization can pay off in reducing costs of fabrication—and it may be a necessity to move manufacturing to a foundry to achieve those low costs.
Another crucial aspect of packaging is getting light onto and off a photonic chip reliably and efficiently, which generally requires fibers. In the long term, it would be ideal to reduce the cost to that of attaching fibers to that of wire bonding, but that remains far off. Fiber bandwidth is high enough that three or four fibers can replace 50 wire connections. We may think fiber cores are small at 8 to 10 μm, but the typical waveguides in a photonic integrated circuit (PIC) are only 1 µm, making precision fiber coupling crucial.
Applications
Telecommunications applications were the first to use silicon photonics, and they continue to be prominent. Datacenters are huge and need to move tremendous amounts of energy as well as information. Integrating the photonics and bringing them closer to the datacenter can reduce energy use and increase communications efficiency.
Brown says many people have come to his lab recently looking for integrated photonics for applications in the quantum world. They need reliable, low-loss photonic waveguides and tiny quantum light sources for emerging applications in communications and computers.
Photonic integration also is hot in other emerging applications, including lidar for autonomous vehicles and other types of sensing. Neural networks and artificial intelligence require high levels of interconnections and processing. Many of these applications require extensive information processing and would seem natural candidates to merge their photonic networks with electronic information processing to improve performance.
Yet, foundry-style processing optimized for low cost and high volume brings tradeoffs for integrated photonics. Optimizing for cost and volume requires rigid rules for packaging and design of features such as connection layout and fiber connections. Operators don’t want to risk costly contamination by allowing new materials into large facilities. Metalenses are flat and use free-space optics, so large-scale foundries are not ready yet, according to Brown. But developers have found smaller foundries to produce some components.
REFERENCES
1. S. E. Miller, Bell Sys. Tech. J., 48, 7, 2059–2069 (Sep. 1969); doi.10.1002/j.1538-7305.1969.tb01165.x.
2. L. Berlin, The Man Behind the Microchip: Robert Noyce and the Invention of Silicon Valley, Oxford University Press (2005).
3. See http://bit.ly/Hecht-Jun21Ref3.
4. See http://bit.ly/Hecht-Jun21Ref4.
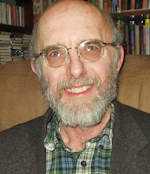
Jeff Hecht | Contributing Editor
Jeff Hecht is a regular contributing editor to Laser Focus World and has been covering the laser industry for 35 years. A prolific book author, Jeff's published works include “Understanding Fiber Optics,” “Understanding Lasers,” “The Laser Guidebook,” and “Beam Weapons: The Next Arms Race.” He also has written books on the histories of lasers and fiber optics, including “City of Light: The Story of Fiber Optics,” and “Beam: The Race to Make the Laser.” Find out more at jeffhecht.com.