PHOTONIC FRONTIERS: ULTRAFAST LASER PROCESSING: Ultrafast lasers make ultraprecise tools
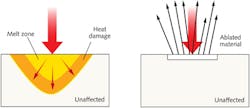
Improved technology has helped ultrafast lasers move beyond the laboratory into the worlds of industry and medicine, where they can perform precise and delicate operations. Their success comes from their ability to concentrate light energy into an interval of picoseconds to femtoseconds, and focus that light onto a small volume of space. That concentration provides the high intensity needed to ablate material from the surface quickly and cleanly, without damaging the underlying areas.
This combination of precision and delicacy is invaluable for applications such as machining brittle materials like glass or ceramics, or making clean holes in hard metals like turbine blades. It also has earned ultrafast lasers a niche in medicine, both for fabricating delicate biomedical devices, such as the stents used in coronary bypass surgery, and in performing sensitive medical procedures such as corneal surgery.
Laser-material interactions
Power intensity and pulse duration are key factors influencing how laser beams interact with materials. Over long time scales, a material absorbs a fraction of the light energy, converting it into heat, which can be conducted through the material. If the beam is powerful enough, it melts the material, and the molten material conducts heat to surrounding areas. Absorption, melting, and heat conduction dominate for time scales as short as nanoseconds.Things change significantly when the pulse energy is delivered on time scales shorter than about 100 ps, with the transition depending on the material. As the peak power of the pulse rises, the peak intensity rises sharply. For example, microjoule pulses lasting 1 ps have a peak power of 1 MW and-when focused to a 5 μm spot, can produce peak intensities of about 4 × 1012 W/cm2, enough to rip off the outer-shell electrons. The interactions are so fast that the ions are ablated from the surface before they can transfer energy to the underlying material. Energy transfer in this ablative mode depends less on material absorption than at lower intensities, but does vary among materials and with laser wavelength. For example, ultraviolet pulses cut transparent materials like glass better than near-infrared pulses, which glass transmits better.
The result is sometimes called "cold ablation." Although the surface briefly becomes very hot, the ions are ablated before they can heat or damage the underlying layers, as shown in Fig. 1. Thus picosecond or femtosecond pulses can remove very thin layers from delicate or fragile materials without damage. Polish researchers have used 70 ps pulses with intensity just above the ablation threshold to remove transparent varnish from oil paintings, using optical coherence tomography to monitor removal.1 Picosecond lasers can write patterns onto the head of a match without igniting it, says Joyce Kilmer of Photonics Industries (Bohemia, NY).
In general, picosecond ablation tends to be faster because the pulses usually carry more energy, but femtosecond ablation tends to produce smoother, more precise surfaces. The actual performance depends on pulse parameters, target material, and other considerations. Repetition rate is important because ablation blasts a plume of material into the path of the beam. Megahertz repetition rates may not leave enough time for the plume from one pulse to dissipate before the next one is fired. Kilohertz repetition rates allow time for that material to dissipate, so they may be more effective in removing material precisely.
Materials working
The cold ablation process can be used for a wide range of materials, including metals, semiconductors, glasses, crystals, and ceramics. Typical thresholds for cold ablation range from 0.05 to 5 J/cm2, as shown in the table for femtosecond pulses from ytterbium-fiber and Ti:sapphire lasers.2
Ultrashort pulses are particularly attractive for cutting or machining brittle materials, including glass, ceramics, silicon, and CIGS (copper-indium-gallium selenide, used in thin-film solar cells). The ablation process avoids cracks when drilling holes or cutting glass, producing sharp, clean edges and surfaces, as shown in Fig. 2. Thin glass plates used in liquid-crystal displays or on cell phones can be cut to shape by mechanically stressing them along a series of cold-ablation laser-drilled holes. Because ablation is a nonlinear process with a high threshold, pulses that are focused so laser power exceeds the ablation threshold only at the center of the focal spot can drill holes smaller than the diffraction limit.Process dynamics can be complex for thin films, such as silica on silicon. Instead of ablating silica from the top down, picosecond pulses penetrate the transparent silica to melt the silicon, then evaporate enough of the melt to lift the thin film up from the substrate, reported Sonja Hermann of the Institute for Solar Energy Research Hameln (Emmerthal, Germany) and colleagues. Thus the threshold depends on the SiO2 thickness.3
Picosecond pulses focused with high-numerical-aperture optics can etch features inside glass or other transparent materials without affecting the surface, because power density is much higher at the focus spot. For example, Benye Li of the Beijing Institute of Technology (Beijing, China) and colleagues wrote a long-period fiber Bragg grating across the core of a singlemode fiber with 35 fs pulses from a Ti:sapphire laser.4 The grating produced 20 dB attenuation in the 1465–1575 nm band. By inducing birefringence in fused silica waveguides inside bulk glass, Luís Fernandes of the University of Toronto (Toronto, ON, Canada) and colleagues made 2-cm-long wavelength-selective directional couplers with extinction ratios to 24 dB. They wrote that the splitters "promise to open new directions for creating polarization-dependent devices in three dimensional optical circuits."5
Nanoparticles and nanofibers
Ultrafast lasers also offer a new twist to pulsed laser deposition. Nanosecond laser pulses have been standard for thin-film deposition, but they can splash 10 μm droplets onto the film. When Inam Mirza and James G. Lunney of Trinity College Dublin (Dublin, Ireland) examined the flux that nanosecond pulses produced from silver targets, they found that the ion flux exceeded the deposition rate, indicating some self-sputtering at the surface. However, they found the ion flux produced by femtosecond pulses was only 1% of the deposition rate, indicating that most of the ablated material formed nanoparticles.6 Other studies show that size of the nanoparticles produced by femtosecond pulses depends on the laser flux, gas environment, and target materials.
Femtosecond pulses easily produce dense tangled masses of silica nanofibers, report Krishnan Venkatakrishnan and colleagues of Ryerson University (Toronto, ON, Canada). Focusing a 12.4 MHz train of 214 fs pulses from a Yb fiber to an intensity of 1.17 J/cm2 on silicon produced four types of nanofilaments. The largest were hundreds of nanometers in diameter and as long as 10 mm. The finest fibers were tens of nanometers in diameter and stretched to hundreds of microns. However, the tangling makes study difficult.7
Medical applications
The ability of ultrafast pulses to cut cleanly without damaging surrounding areas or forming rough edges is vital both for processing medical implants and for delicate surgery.
Smooth surfaces are particularly important in stents, expandable tubes that are inserted into clogged arteries and opened to restore blood flow. The body sometimes reacts to the implant by coating the stent with scar tissue, which can re-clog the artery. Machining stents made of various materials with ultrafast lasers produces surfaces so smooth that they reduce the chance of scar tissue growth.
Femtosecond lasers also have become a standard tool for cutting flaps in the surface of the cornea to expose the interior for LASIK refractive surgery. A major attraction is its ability to cut the flaps more accurately than conventional surgery.
Now ophthalmologists are expanding femtosecond laser techniques to cataract surgery. One goal has been softening the hardened core of the lens that caused the cataract, so it can be removed easily. Another is performing the incisions needed to remove the lens and insert a replacement with minimal damage to other parts of the eye. Three companies are developing femtosecond laser systems for both processes.8
Results so far are encouraging. At the American Academy of Ophthalmology meeting in October, William Culbertson of the Bascom Palmer Eye Institute at the University of Miami School of Medicine (Miami, FL) reported that femtosecond laser treatment eased the surgical requirements and reduced ultrasound exposure during lens removal. At the same meeting, Mark Packer of the Oregon Health and Sciences University (Portland, Oregon) reported that the femtosecond laser surgery avoided the loss of critical corneal endothelial cells, which can be damaged in conventional cataract surgery.
Outlook
Ultrafast processing owes much to the industrialization of picosecond and femtosecond lasers so nonspecialists can use them in industrial and medical environments. So far it has been most successful in niche applications, and cost and the rate of material removal remain significant limitations. However, the performance advantages can be compelling for demanding applications, such as stents and delicate eye surgery.
REFERENCES
1. P. Targowski et al., "Picosecond laser ablation system with process control by Optical Coherence Tomography," Proc. SPIE, 7391, 7391-15 (2009).
2. IMRA, Technical Note T-0401, "High Precision Machining"; http://www.imra.com/pdf/TechNoteT0401.pdf.
3. S. Hermann et al., "Picosecond laser ablation of SiO2 layers on silicon substrates," Appl. Phys. A, 99, 151–158 (2010); doi:10.1007/s00339-009-5464-z.
4. B. Li et al., "Femtosecond laser fabrication of long period fiber gratings and applications in refractive index sensing," Optics & Laser Technol., 43, 1420–1423 (2011).
5. L.A. Fernandes et al., "Femtosecond laser fabrication of birefringent directional couplers as polarization beam splitters in fused silica," Opt. Exp., 19, 11992 (June 20, 2011).
6. I. Mirza and J.G. Lunney, "Fabrication of metal nanoparticle films using pulsed laser deposition," 30th ICPIG, Belfast, Northern Ireland, UK (Aug. 28–Sept. 2, 2011); http://mpserver.pst.qub.ac.uk/sites/icpig2011/187_D13_Mirza.pdf.
7. K. Venkatakrishnan et al., "Nanofibre fabrication by femtosecond laser ablation of silica glass," Opt. Exp., 19, 15776 (Aug. 15, 2011).
8. L. He, K. Sheehy, and W. Culbertson, "Femtosecond laser-assisted cataract surgery," Current Opinion in Ophthalmol., 22, 43–52 (January 2011); doi:10.1097/ICU.0b013e3283414f76.
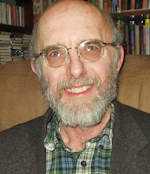
Jeff Hecht | Contributing Editor
Jeff Hecht is a regular contributing editor to Laser Focus World and has been covering the laser industry for 35 years. A prolific book author, Jeff's published works include “Understanding Fiber Optics,” “Understanding Lasers,” “The Laser Guidebook,” and “Beam Weapons: The Next Arms Race.” He also has written books on the histories of lasers and fiber optics, including “City of Light: The Story of Fiber Optics,” and “Beam: The Race to Make the Laser.” Find out more at jeffhecht.com.