Alkali metals improve efficiency of perovskite solar cells
Perovskite-based solar cells are simple and cheap to produce, offer flexibility that could unlock a wide new range of installation methods and places, and in recent years have reached energy efficiencies approaching those of traditional silicon-based cells. But figuring out how to produce perovskite-based energy devices that last longer than a couple of months has been a challenge.
Now researchers from Georgia Institute of Technology (Georgia Tech; Atlanta, GA), University of California San Diego (UCSD; San Diego, CA) and Massachusetts Institute of Technology (MIT; Cambridge, MA) have reported new findings about perovskite solar cells that could lead the way to devices that perform better.
"Perovskite solar cells offer a lot of potential advantages because they are extremely lightweight and can be made with flexible plastic substrates," said Juan-Pablo Correa-Baena, an assistant professor in the Georgia Tech School of Materials Science and Engineering. "To be able to compete in the marketplace with silicon-based solar cells, however, they need to be more efficient."
In a study that was published in the journal Science and sponsored by the U.S Department of Energy (DoE) and the National Science Foundation (NSF), the researchers described in greater detail the mechanisms of how adding alkali metal to traditional perovskites leads to better performance. The paper is entitled "Homogenized halides and alkali cation segregation in alloyed organic-inorganic perovskites" from Juan-Pablo Correa-Baena et al., Science (February 2019) at http://dx.doi.org/10.1126/science.aah5065.
"Perovskites could really change the game in solar," said David Fenning, a professor of nanoengineering at UCSD. "They have the potential to reduce costs without giving up performance. But there's still a lot to learn fundamentally about these materials."
To understand perovskite crystals, it's helpful to think of its crystalline structure as a triad. One part of the triad is typically formed from the element lead. The second is typically made up of an organic component such as methylammonium, and the third is often comprised of other halides such as bromine and iodine. In recent years, researchers have focused on testing different recipes to achieve better efficiencies, such as adding iodine and bromine to the lead component of the structure. Later, they tried substituting cesium and rubidium to the part of the perovskite typically occupied by organic molecules.
"We knew from earlier work that adding cesium and rubidium to a mixed bromine and iodine lead perovskite leads to better stability and higher performance," Correa-Baena said. But little was known about why adding those alkali metals improved performance of the perovskites.
To understand exactly why that seemed to work, the researchers used high-intensity X-ray mapping to examine the perovskites at the nanoscale. "By looking at the composition within the perovskite material, we can see how each individual element plays a role in improving the performance of the device," said Yanqi (Grace) Luo, a nanoengineering PhD student at UCSD.
They discovered that when the cesium and rubidium were added to the mixed bromine and iodine lead perovskite, it caused the bromine and iodine to mix together more homogeneously, resulting in up to 2% higher conversion efficiency than the materials without these additives. "We found that uniformity in the chemistry and structure is what helps a perovskite solar cell operate at its fullest potential," Fenning said. "Any heterogeneity in that backbone is like a weak link in the chain."
Even so, the researchers also observed that while adding rubidium or cesium caused the bromine and iodine to become more homogenous, the halide metals themselves within their own cation remained fairly clustered, creating inactive "dead zones" in the solar cell that produce no current. "This was surprising," Fenning said. "Having these dead zones would typically kill a solar cell. In other materials, they act like black holes that suck in electrons from other regions and never let them go, so you lose current and voltage. But in these perovskites, we saw that the dead zones around rubidium and cesium weren't too detrimental to solar cell performance, though there was some current loss," Fenning said. "This shows how robust these materials are but also that there's even more opportunity for improvement." The findings add to the understanding of how the perovskite-based devices work at the nanoscale and could lay the groundwork for future improvements.
"These materials promise to be very cost effective and high performing, which is pretty much what we need to make sure photovoltaic panels are deployed widely," Correa-Baena said. "We want to try to offset issues of climate change, so the idea is to have photovoltaic cells that are as cheap as possible."
This research was supported by the U.S. DoE EERE postdoctoral fellowship and grant Nos. DE-SC0001088 and DE-AC02-06CH11357, the California Energy Commission under grant No. EPC-16-050, the Skoltech NGP Program under grant No. 1913/R, the Hellman Fellowship and the NSF under grant Nos. CBET-1605495, DMR-1507803, GRFP 1122374, CHE-1338173 and ECCS-1542148.
SOURCE: Georgia Tech; http://www.rh.gatech.edu/news/617145/researchers-chart-path-cheaper-flexible-solar-cells
About the Author
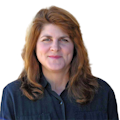
Gail Overton
Senior Editor (2004-2020)
Gail has more than 30 years of engineering, marketing, product management, and editorial experience in the photonics and optical communications industry. Before joining the staff at Laser Focus World in 2004, she held many product management and product marketing roles in the fiber-optics industry, most notably at Hughes (El Segundo, CA), GTE Labs (Waltham, MA), Corning (Corning, NY), Photon Kinetics (Beaverton, OR), and Newport Corporation (Irvine, CA). During her marketing career, Gail published articles in WDM Solutions and Sensors magazine and traveled internationally to conduct product and sales training. Gail received her BS degree in physics, with an emphasis in optics, from San Diego State University in San Diego, CA in May 1986.