Molecular imaging is proving to be a boon to drug development. “The whole pharmaceutical industry has become more interested in imaging,” says Barbara Y. Croft, Ph.D, of the Cancer Imaging Program (CIP) at the National Cancer Institute (Bethesda, MD). “They sense there’s something here they are not utilizing as well as they could.”
The CIP funds research that helps to drive development of instrumentation and its application for the management of cancer and cancer risk. Traditional imaging methods are limited in terms of sensitivity, resolution, and ability to track disease development over time. Croft explains that molecular imaging, an emerging field that allows noninvasive visualization of biological processes in vivo, enables testing at intervals to illustrate how tumors develop and respond to drugs. Molecular imaging depends on the use of specific molecules (probes) that carry reporters—for instance, positron-emitting radio-isotopes readable by positron emission tomography (PET)—and are able to selectively target these biological processes.
Because the area of molecular imaging is so new, it is not broadly understood—and last year, the Society for Nuclear Medicine advanced a professional definition to help clarify. “Molecular imaging is the visualization, characterization, and measurement of biological processes at the molecular and cellular levels in humans and other living systems,” the SNM said. “Molecular imaging typically includes two- or three-dimensional imaging as well as quantification over time. The techniques used include radiotracer imaging/nuclear medicine, MRI, MRS, optical imaging, ultrasound and others.”
The ability to see the dynamic effect of drugs at cellular and subcellular levels is important to understanding their operation and potential. Croft emphasizes the importance of instrumentation advances in disease management. Getting instruments and information into the hands of more people, she says, will speed the drug development process, saving critical time and expense—and thus expand impact.
According to Stephen Oldfield, director of imaging marketing at Caliper Life Sciences, maker of the IVIS molecular imaging systems, the systems shortens the optimization process. “Success in drug development is about cycles of optimization,” Oldfield says. “With molecular imaging techniques you can get compounds into animals sooner and see the results faster—so you get around the optimization cycle more times. Molecular imaging also supports a wider variety of biological models to answer complex questions.” He added, “Our customers calculate their savings in FTE time and in fewer animals used, but they describe the additional insights they gain as ‘priceless.’”
Markus Lusser, vice president of sales and marketing for the Siemens Medical Solutions Nuclear Medicine Group, agrees. Molecular imaging, he says, is “incredibly important for the pharmaceutical companies, and many of them are investing heavily in imaging programs that also have very sophisticated biomarkers associated with them.”
Instrumentation advantage
As if to illustrate the point, GlaxoSmithKline (GSK) opened its new Clinical Imaging Centre in June at the Hammersmith Hospital in London, England. The largest European imaging center dedicated to clinical research, the center was funded by GSK with a capital investment of £46 million (US$85.3 million, and GSK partners ponied up another £37 million (US$66.7 million) to build it. Plus, GSK has endowed it with £11 million (US$20.4 million) in annual operating funds. The center includes a radiochemistry facility, and PET and MRI scanners. GSK has attracted several “physician-scientists” to staff the center, including Edward Bullmore, who continues on half time at Cambridge University as professor of psychiatry. Bullmore has conducted early, exploratory work on depression that GSK says, “points toward where imaging may take pharmaceutical development by revealing the underlying biology of illness until now defined mostly by its clinical symptoms.”
Another impressive facility, the Center for Molecular Imaging Research (CMIR) is run jointly by the Massachusetts General Hospital (MGH) and Harvard Medical School. The center is run by a team of experts drawn from three areas: physicians and technicians, chemists and biochemists, and physicists and imaging specialists. One of these experts is Matthias Nahrendorf, MD PhD, of the MGH Center for Systems Biology, who explains that CMIR gets the vast majority of its funding from the National Institutes of Health, plus additional support from private foundations and industry. But “our customers are the big pharma companies,” says Dr. Nahrendorf. “They come by to look at our facility, and then model their own facilities after it,” he explains. The CMIR’s Mouse Imaging Program uses several state-of-the-art in vivo imaging technologies: high-resolution magnetic resonance (MR), positron emission tomography (PET), single-photon-emission computed tomography (SPECT), computed tomography (CT), fluorescence mediated tomography (FMT), bioluminescence, various fluorescence imaging technologies and intravital confocal microscopy (see Fig. 1). The integrated program also provides mouse holding facilities for serial imaging, surgery, anesthesia, veterinary care and imaging agents. Image reconstruction, 3-D display, fusion, quantitative image analysis, and server access are also available.
Molecular imaging is important because it reveals not just the anatomy—for example, the size of a cancer—but biology, says Nahrendorf. Because of this, it enables earlier detection and greater efficacy of treatment. “Previously,” he says, “an initial CT would measure the size of a tumor. The patient would receive therapy and we’d find out six months later whether the therapy was successful. We’d take another CT and measure the tumor and begin again. But with molecular imaging, we can tell one to two weeks into treatment whether the therapy is working, and if not, switch to something else.”
Siemens’ Lusser cites a recent study that demonstrated physicians changed their intended management in 36.5% of cases when give the additional advantage of using PET technology.1
Nahrendorf notes that there has been no good clinical tool for cardiovascular assessment to determine the risk of stroke or infarct—and says that 50% of all infarcts are triggered by things that are generally considered unimportant by current methods such as stress tests and catheters. A paper Nahrendorf coauthored demonstrates the use of a novel technology, fluorescence mediated tomography (FMT), to address this problem.2 FMT enables molecular sensing in intact organisms in vivo—and by the way, the technology is an example of results that the CMIR has produced. FMT, which is being commercialized by VisEn Medical, produces three-dimensional images using near-infrared fluorescent molecular beacons and inversion techniques that take into account the diffuse nature of photon propagation in tissue. “With fluorescence reflectance there is a disadvantage,” says Nahrendorf, “you don’t know what’s happening inside the mouse. It’s very superficial, and not quantitative.” FMT, by contrast, adds the third dimension and quantifies the information. Enzyme-activatable fluorochromes can be detected with high positional accuracy in deep tissues, molecular specificities of different beacons toward enzymes can be resolved, and tomography of beacon activation is linearly related to enzyme concentration. The tomographic imaging method promises a range of new capabilities for studying biological function; for example, identifying molecular-expression patterns by multispectral imaging or continuously monitoring the efficacy of therapeutic drugs.
The importance of probes
Nahrendorf says much work is being done to advance the development of probes—for instance near-IR fluorochromes that have optimized pharmacokinetics and can reflect in specific wavelengths, nanoparticle tags with affinity for ligands and peptides, and an activatable class of agents that fluoresce only when they come in contact with a target. In fact, Nahrendorf and a group of colleagues have worked to develop an agent to enable real-time detection of vascular cell adhesion molecule-1 (VCAM-1) expression in experimental atherosclerosis in vivo (see Fig. 2). Further, the agent can quantify pharmacotherapy-induced reductions in VCAM-1 expression, and identify activated cells in human plaques. This clinically translatable agent could noninvasively detect inflammation in early, subclinical atherosclerosis.This work is one step toward overcoming what Nahrendorf identifies as a major hurdle: translation from preclinical to clinical application. To be able to use probes in a human patient, the probes must have low toxicity and high sensitivity. “Our work in this area is ongoing,” he says.
Charles Manning, Ph.D., of Vanderbilt University’s Institute of Imaging Sciences (VUIIS), is a member of the Task Force for Optical Imaging within SNM’s Molecular Imaging Center of Excellence. “A lot of what we do in our research involves imaging agents,” he says. While he and his colleagues “can rapidly interrogate biological factors or physiological response quite efficiently,” he also says that one of the challenges in designing molecular probes is specificity for the target of interest.
This is especially important when there are confounding issues, he says, describing a situation in which a probe he was using had good specificity and sensitivity, but the results of an experiment were not as clear as hoped because the probe he was using was known to express on inflammation cells—and the model had inflammation.
“To go from target of interest to new chemistry is a very difficult process,” he says, explaining that development of new probes is “sort of a bottleneck.” He notes that there’s a lot of potential synergy with technologies such as high-throughput screening, used for drug discovery. “At Vanderbilt we’re lucky to have a wonderful high-throughput screening facility,” he says. “As those types of facilities start to move into the academic realm, we ask, ‘can these tools be used not for drug compounds at all but to discover new imaging probes?’ As we get better reagents that are increasingly specific we can better understand what is happening.” And while the task is not easy, he’s optimistic, given the extensive libraries and technologies available to be explored. “The future is bright,” he says.
Preclinical to clinical
David Steinberg of Enlight Biosciences echoes Nahrendorf when he says, “There’s a big disconnect between preclinical and clinical.” Enlight is a biotech research firm launched this year by PureTech Ventures and several major pharmaceutical companies, and cofounded by such academic luminaries as Nobel Laureate Dr. H. Robert Horvitz of MIT. The company announced in July that it will direct up to $39 million “to advance breakthrough technologies that can fundamentally alter drug discovery and development.” Steinberg says the company will focus on technologies that will “connect preclinical research, clinical development, and medical practice.”
Enlight has already initiated programs in molecular imaging—as well as biologics and drug delivery. The work is done in a precompetitive model; Merck, Pfizer, and Eli Lilly, as members, have the opportunity to invest in the development.
“PureTech Ventures exists to help commercialize academic innovations for unmet needs in life sciences,” says Steinberg. PET is an example of a technology that has vitally enabled pharmaceutical development, but commercialization of new instrumentation has lagged. “We noticed a steep decline in recent years in venture-backed companies working on tool development,” Steinberg notes. Most traditional life-science investors have focused instead on late-stage therapeutic programs. As a consequence, important technologies that could be of great strategic impact to the pharmaceutical industry are not being commercialized.
“We’re working to enable a process whereby pharmaceutical companies can have continuity from preclinical to clinical,” says Steinberg. Being able to use the same marker and a complementary modality is very important.
References
- B.E. Hillner et al., J. Clinical Oncology 26 (2006)
- M. Nahrendorf et al., Circulation Research, (March 22, 2007)
About the Author
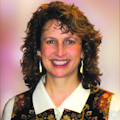
Barbara Gefvert
Editor-in-Chief, BioOptics World (2008-2020)
Barbara G. Gefvert has been a science and technology editor and writer since 1987, and served as editor in chief on multiple publications, including Sensors magazine for nearly a decade.