BIOMEDICAL IMAGING/LASER TISSUE INTERACTION: LAMMP lights the way from bench to bedside
Inside a 37,000 square-foot facility on the campus of the University of California, Irvine, laser light courses through a fiber-optic probe and hits its mark: a tumor recently doused with anti-cancer drugs. Based on the light scatter patterns within the tumor, researchers can judge the effectiveness of the therapy to shrink the tumor. This is just one example of the biophotonics experiments performed by researchers at the Laser Microbeam and Medical Program (LAMMP), a Biotechnology Resource Center funded by the National Center for Research Resources.
A combination of unique facilities (LAMMP has an on-site operating room and is part of the Beckman Laser Institute and Medical Clinic [BLI]) and creative research staff has given LAMMP a strong track record of light alchemy: transforming promising fundamental discoveries into application-ready clinical technologies. Led by Bruce Tromberg, an enthusiastic advocate for balancing bench and bedside needs, LAMMP supports technology development at all spatial scales from cells to human subjects. Throughout his discussions about LAMMP, Tromberg stresses that what happens at LAMMP is really very simple: discovering different ways to modulate light in space, time and wavelength.
He also stresses the importance of moving freely between basic research and clinical work to better understand the mechanisms that drive clinical outcomes. Each of the more than 40 ongoing clinical protocols at BLI that involve LAMMP technology has a corresponding laboratory study to examine the cellular and molecular drivers that underpin the clinical work. “It’s important to keep the connection between medicine and biological mechanisms,” says Tromberg.
To illustrate the connection between fundamental research and clinical work Tromberg describes a cauldron filled with an optics and photonics alphabet soup. “We are constantly stirring the soup and sometimes the letters come together to form acronyms. These become devices and systems. The pot is the foundation. Without it we have no translation, no clinical application.”
From cauldron to clinic
One very successful soup creation is the dynamic cooling device (DCD) developed in the laboratory of BLI Medical Director J. Stuart Nelson. The original research examined how to cool biological tissue as it interacts with laser light. Further studies led to a patented clinical technology to cool skin undergoing laser treatment for port wine stains, a purplish birthmark. Licensed by Candela (Wayland, MA), the technology is now a component on 18,000 lasers around the world and used in a number of cosmetic procedures such as laser hair removal.
A second soup creation, DOSI—diffuse optical spectroscopic imaging—is just making its clinical debut. In April of this year, LAMMP announced that the American College of Radiology Imaging Network (ACRIN) would oversee a five-site clinical trial for breast cancer monitoring using DOSI, a technology Tromberg began working on 20 years ago. DOSI tracks near-infrared light as it scatters through a breast tumor. The scatter patterns reveal information about the tumor’s hemoglobin, water and fat content. Researchers can use this information to determine how well medications given before surgery are working to shrink a tumor. DOSI provides an advantage over some current imaging modalities because it can be done in a doctor’s office on a frequent basis, is inexpensive and noninvasive.
The trial will enroll 60 patients and use a portable, refrigerator-size system with an extendable handpiece to noninvasively monitor tumor uptake of anti-cancer drugs. Trial sites are: Dartmouth, Harvard/Massachusetts General Hospital, the University of Pennsylvania, the University of California, San Francisco, and the University of California, Irvine.
Tromberg notes that the trial is important because “We haven’t had any classic clinical trials in breast cancer using optical technology. The field needs a non-anecdotal trial and we [have a technology] that can show the way.”
Virtual modeling
In addition to DOSI and other core technologies such as multi-dimensional and spatially modulated microscopy, microendoscopy/optical coherence tomography, and wide field functional imaging, LAMMP has developed a modeling and analysis component to strengthen the computational side of imaging.Now in its fourth year, the Virtual Photonics Technology Lab (VPTL) offers a virtual computing environment with open source code for experts and those new to biooptics. For biooptics researchers who may not have access to modeling software, but need to simulate light interactions with tissue, VPTL provides a suite of tools to perform basic biooptics measurements. For individuals with little or no biooptics experience, VPTL offers training on the VPTL software and on the fundamentals of light transport in tissue.
Tromberg notes that some of the VPTL modeling software could also be used to help define novel technologies to solve clinical problems. For instance, surgeons interested in locating specific nerves during surgery could work with LAMMP faculty to simulate forceps containing fiber optic probes. The software might indicate optimal wavelength choices for the probes or provide spatial information to ensure image formation within the device.
As part of its educational outreach efforts, LAMMP organizes an annual workshop that includes hands-on demonstrations of the lab’s software and invited talks from modeling experts who discuss computational issues in biooptics. This year’s meeting will be held August 19–20 at the University of California, Irvine.
Between its online presence and annual workshop VPTL’s researchers hope to “ensure a larger population has the rudimentary understanding [of light/tissue interactions] to perform quantitative processing of biooptics data,” says Vasan Venugopalan, one of VPTL’s principal investigators. “We want to raise the bar across the field on how to use modeling.”
Toward Maxwell’s equations
Tromberg and his LAMMP colleagues will continue to spur growth of biomedical optics as they identify clinical problems and discover how to apply biooptics to fundamental problems. He notes that optics now is helping to stimulate an interest in physiology and metabolism. In the near future, Tromberg foresees metabolism studies, especially the regulation of metabolic pathways, as a critical research area.
“Biology has the equivalent of Maxwell’s Equations*, but we don’t know what they are yet. It’s extremely important that we keep hammering away at these,” says Tromberg. “We must continue to search for fundamental phenomena and discover how they impact disease across spatial scales.”
* The equations that explain the fundamentals of electricity and optics.
About the Author
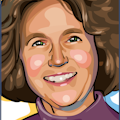