LATTICE LIGHT-SHEET MICROSCOPY: Laureate's reprise shows real-time subcellular activity in 3D
As the Nobel Committee for Chemistry was announcing the 2014 prize would go to Eric Betzig (and Stefan Hell and W. E. Moerner; see "Nobel Prize honors super-resolution microscopy pioneers"), Betzig and colleagues at the Howard Hughes Medical Institute's Janelia Research Campus were preparing to announce a publication describing lattice light-sheet microscopy, a new technique they developed to quickly collect high-resolution images while minimizing damage to cells.1 The approach captures the three-dimensional (3D) activity of molecules, cells, and embryos in fine detail over longer periods than was previously possible.
The Nobel Laureate wants the technique to be widely used, even as technology development continues in his own lab. His team has built a second microscope for Janelia's Advanced Imaging Center, where it will be available to visiting scientists free of charge (scientists wanting to use it can submit a proposal at www.janelia.org/aic), and has deployed two more to labs at Harvard University (Cambridge, MA) and the University of California, San Francisco.
Development of the approach
Imaging at high resolution in 3D has typically meant sacrificing speed and subjecting cells to significant light-induced toxicity. The new design evolved from one Betzig unveiled in 2011, which illuminates samples with a virtual sheet of light, created when a beam of non-diffracting light (a Bessel beam) sweeps across the imaging field.2 It produces high-resolution images, imposes less photodamage than a traditional microscope, and is fast enough to record dynamic processes in living cells.
In designing the Bessel beam system, Betzig's team had to address a problem related to the beam's shape. "It's not just a thin pencil of light—it has these dimmer side lobes," Betzig explains. "So when you sweep that across a sample, you get out-of-focus light." One solution was to shift the beam incrementally, illuminating the specimen with a grating pattern. The researchers could then computationally strip out the blurriness caused by the side lobes-a technique known as structured illumination.
Structured illumination can also help overcome light microscopy's spatial resolution limits. Applying a technique developed by Mats Gustafsson, the team moved the Bessel beam to produce a lattice-like light pattern. That removed the side lobe problem and pushed the resolution a bit beyond the diffraction limit.
To reduce the time required to move the Bessel beam each time a sample was imaged, the developers split the beam into seven parallel parts, so each traveled just one-seventh of the original distance. Interestingly, that decreased phototoxicity, too.
Could they reduce light toxicity even more by dividing the Bessel beam? Betzig wasn't sure what would happen when the beams began to crowd one another, so he modeled the potential effects. The model proposed certain arrangements where that interference actually destroyed the undesirable side lobes.
Then Betzig resurrected a decade-old theory he'd proposed and named optical lattice microscopy; it would limit damage by illuminating a sample with a massive 3D array of light foci. The lattice theory allowed the team "to predict the patterns that would produce these magic periods," Betzig says.
Moving from theory to reality proved challenging, but postdoctoral researchers Bi-Chang Chen and Kai Wang devised a way to create the optical lattice by modulating light directly on the imaging plane. Months of work produced 3D images of cells with the detail, speed, and low toxicity that they'd hoped for.
Working with the system
The new microscope operates in two modes. One uses the principles of structured illumination to create very high-res output by collecting and processing multiple images of every plane. Imaging can be sped up to capture faster processes (albeit at lower resolution) with an alternative "dithered" mode, which lowers light exposure, and thus damage to cells; in many cases, tagged proteins are naturally replaced by cells before their signal fades appreciably. "So there are many cells you could look at forever in 3D," Betzig says.
Chen, Wang, and fellow postdoc Wesley Legant have helped optimize the technology for a variety of experiments. "This is not a single imaging technique," Wang says. "It's an imaging platform."
The microscope's high resolution enables tracking of individual proteins in 3D, and these applications are among the most exciting, Betzig says. "Normally when people do single-molecule studies, they have to do them in thin, flat cells, because the out-of-focus light kills you if it's thick." The lattice's ultra-thin light sheet eliminates that problem and permits single molecules to be seen, even in large multicellular specimens. The microscope is also fast enough to track the rapid growth and retraction of cytoskeletal components in dividing cells, and gentle enough to monitor the molecular dynamics of developmental processes that unfold over many hours.
After testing the technique at Janelia, Harvard cell biologist Tomas Kirchhausen worked with Betzig's team to purchase the parts and assemble a "clone" (Betzig's lab freely shares its designs and provides instructions to those with the expertise to build their own). Kirchhausen's team is now generating movies of dynamic processes in living cells (tracking the assembly of thousands of vesicles, watching viruses enter cells, and studying how cells change size as they divide), observing such processes in a more complete, 3D context instead of seeing just one plane at a time.
Zeiss has licensed the Bessel beam and lattice light-sheet technology. "Ultimately, commercialization is the crucial last step to ensuring that these technologies can have broad impact in the research community," Betzig says.
1. B. C. Chen et al., Science, 346, 6208, 1257998 (2014); doi:10.1126/science.1257998.
2. T. A. Planchon et al., Nature Meth., 8, 5, 417–423 (2011); doi:10.1038/nmeth.1586.
About the Author
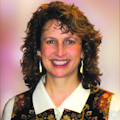
Barbara Gefvert
Editor-in-Chief, BioOptics World (2008-2020)
Barbara G. Gefvert has been a science and technology editor and writer since 1987, and served as editor in chief on multiple publications, including Sensors magazine for nearly a decade.