BIOMEDICAL IMAGING: National Institute's imaging research collaborates for effect
Several optical imaging researchers at the National Institute of Biomedical Imaging and Bioengineering (NIBIB) are part of a new wave of investigators pressing new materials and designs into service to create windows into the sub-cellular world of DNA, neural transmission and chemical transport. Their work is focused on developing techniques to answer fundamental questions in biology and medicine, but proximity to the National Institutes of Health clinical facilities also enables them to collaborate on healthcare challenges.
The researchers are scattered through three sections of NIH's Building 13: the Biomedical Instrumentation and Multiscale Imaging (BIMI) group, the High Resolution Optical Imaging Section, and the Biophotonics Section. They are among NIH's 6,000 intramural researchers, a small group compared to the more than 300,000 extramural researchers who account for over 80% of the NIH budget.
Macro and nano
While much of the focus of the BIMI group is to create imaging tools for the rest of NIH's intramural programs, the investigators also find time to pursue their own research. Starting out at the macroscopic level, Alexander Gorbach, chief of the Infrared Imaging and Thermometry Unit, applies what he likes to call "infrared photography" to interoperative fields. Gorbach uses infrared cameras to assess blood flow during brain surgery and kidney transplants. He has shown that temperature changes on the cortex are associated with different types of brain tumors, and that IR can predict how well a kidney will function two weeks after surgery. To support his clinical work, Gorbach goes back to the lab to study the mechanisms at work in the clinic. "We move from clinical to animal to see what is actually going on," he says.
Working in the cellular and sub-cellular realms, BIMI colleague Albert Jin, chief of the Nanoinstrumentation and Force Spectroscopy Unit, is collaborating with teams at the University of California–Berkeley and Northwestern University to build a hyperlens nanoscope, which allows researchers to image features down to 160 nm. Jin and another collaborator Ling-Gang Wu, M.D., a neuroscience investigator at the National Institute of Neurological Disorders and Stroke, plan to use the nanoscope to perform high-resolution, high-speed studies of molecular events that occur during synaptic transmission in the brain.
Multimodal imaging
In NIBIB's high-resolution optical imaging lab, microscopist Hari Shroff is collaborating with several teams to allow assessment of proteins and other structures in highly localized areas of cells. Shroff, who previously worked with Eric Betzig at Howard Hughes Medical Institute's Janelia Farms Research Campus, has extended the range of photoactivated localization microscopy (PALM) to provide 3-D super-resolution. Using this new technique, researchers can study a variety of cellular structures such as the mitochondrial network and the nuclear membrane at less than 100 nm axial and less than 50 nm lateral resolution. Collaborating with scientists from Janelia Farms and Florida State University, Shroff has used a genetically expressed fluorescent marker photoactivatable mCherry (PA-mCherry117), to image at depths greater than 8 μm.
Shroff is also developing approaches to improve the resolution of single-plane illumination microscopy (SPIM). In this technique, sheets of light are applied to the specimen at specific intervals, and analysis software creates 3-D images based on the optical sectioning characteristics of the sheet. Shroff is applying SPIM to image C. elegans, a transparent, 1 mm-long worm that was the first multicellular organism to have its genome completely sequenced. His goal is to image in four dimensions the entire 20,000 gene products in C. elegans, which is highly sensitive to light. "We want to get at how DNA gets transformed into an organism," says Shroff. In addition, Shroff sees promise in using SPIM to model neural development in C. elegans—work that could shed light on human neural development.
In addition, he and postdoctoral fellow Andrew York have developed a prototype that can detect the terahertz spectrum given off by skin cancers. Their challenge now is improving resolution to levels comparable with fluorescence microscopy.
Probe imaging
Improving what researchers see using super-resolution techniques is the central focus of the work done by George Patterson, chief of NIBIB's Biophotonics Section. Patterson, who worked with Betzig to create PALM, develops photoactivatable probes to image cell structures. Individual probes can be turned just a few at a time so that each spot imaged represents the signal from a single fluorescent molecule. Because the molecules photobleach rapidly, new molecules that are turned on can be imaged individually and accurately localized. Images from experiments using the photoactivatable probes are created when the coordinates of all the imaged molecules are plotted on a composite image. The new image provides sub-diffraction detail about the locations of each molecule.
Patterson's lab also is applying the probes to cell imaging experiments. In one instance, the probes are part of studies to determine the interaction between viruses and healthy cells. This work could lead to insights that would aid development of effective drug interventions. In a second project, Patterson's group is using super-resolution microscopy to obtain 3-D images of sodium phosphate transporter proteins. If these molecules, which help maintain proper phosphate and calcium levels in the body, are disrupted, conditions such as cardiovascular disease and organ dysfunction can occur.
Beyond imaging
The intramural scientists at NIBIB approach their work from a physical sciences and technological perspective. They try to solve the engineering issues that will improve the tools used to answer fundamental biological and clinical questions. As Albert Jin says, "Deep down, we're scientists and when the technologies we develop are used to solve important problems [related to biology and health], then we are advancing science."
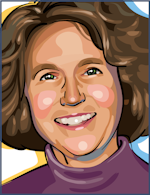