MICROSCOPY/FLUORESCENCE IMAGING/RAMAN SPECTROSCOPY: The hybrid-systems approach to high-power, low-cost microscopy
A new wave of multimodal imaging techniques enables powerful bioimaging at less cost than single-modality approaches, and can deliver more quantitative information. Representative of the trend are a wide-field fluorescence method that combines uniform and structured illumination to create 3-D images of living samples, and a confocal Raman/fluorescence combination involving quantum dots.
Lights, camera, action. Simple as it may sound, that formula drives nearly all microscopy techniques. With the right lighting and a powerful detector, any molecule or cellular process is ready for its close-up. When microscopists achieve just the right balance of illumination, detection, and processing, they are rewarded with a bounty of unprecedented subcellular vistas.
Numerous microscopy approaches now provide reliable insights into biological processes. However, some of these methods carry a high price tag and require specialized optical components and frequent adjustments. A new wave of hybrid microscopy techniques offers the same powerful imaging at a fraction of the cost, and in some cases provides more quantitative information about each sample. Among such techniques are HiLo microscopy and hybrid fluorescence Raman microscopy.
Speckle is key to HiLo
Optical scientists normally try to eliminate noise or at least keep it at extremely low levels. In HiLo microscopy, “noise” created by random speckle patterns is a critical element in the technique’s ability to optically section thick samples. The wide-field fluorescence imaging technique uses a combination of uniform and structured illumination to create 3-D images of living samples, an important advance for optical microscopes. The approach rejects out-of-focus backgrounds and improves contrast of fluorescently labeled structures in thick tissue.
Developed at Boston University (Boston, MA) by Jerome Mertz and his students, the HiLo technique acquires two images—one taken with structured illumination and the other with uniform illumination. Random speckle patterns introduced by an optical diffuser provide the contrast needed to determine whether a portion of the sample under investigation is in focus or not. The random nature of the speckle patterns limits the effects of aberration and scattering on the images.
The HiLo approach uses simple components and processing software to distinguish in-focus signals from an out-of-focus background. After the camera captures fluorescent photons from both in-focus and out-of-focus planes, the analysis program determines which photons come from each plane. The program then subtracts out the out-of-focus photons.
To image through a sample, pairs of images are taken—one with structured illumination and one with uniform illumination. A software program that incorporates a specially designed algorithm systematically interrogates each pair. By fusing the two sets of images, the researchers obtain complete optical sectioning of a sample at high resolution.
Imaging speed depends on the camera and graphic processing unit. Current acquisition rates for live samples range between 10 and 40 fps. Tim Ford, a research assistant in the Mertz lab, notes that as camera rates improve, the acquisition rate will also increase.
Mertz and his colleagues have shown that the images obtained with HiLo microscopy are comparable to those obtained with confocal microscopy. This bodes well for HiLo since confocal microscopy is a highly popular, but costly approach to acquire background-free, optical sectioned samples. Standard confocal microscopes can cost hundreds of thousands of dollars. Their high capital cost often limits their availability to core facilities. “HiLo fills in a gap,” says Ford. “It’s a nice combination of elements that have not been put together before to create a fast, inexpensive, simple-to-implement technique for optical sectioning.”
To provide research laboratories with lower-cost options for optical sectioning, the Mertz group has developed a prototype microscope illuminator that attaches to standard microscopes. The illuminator has two LED sources, one that lights the sample uniformly, and one that lights the sample with a grid structure. The device toggles back and forth between illumination paths at the camera frame rate and processing software displays both the uniform and HiLo images in real time (see Fig. 1). “Labs usually have a cheaper microscope in-house, so with this device they can change it into a sectioning microscope that’s viable,” says Ford.
HiLo microscopy also promises to move into clinical applications. The researchers are working on an endomicroscope that incorporates the HiLo approach. Mertz’s group is working with Satish Singh, M.D., at the Boston University School of Medicine’s Gastroenterology Department. They are applying the technique to optical sectioning of colorectal lesions and Barrett’s esophagus. The HiLo endomicroscope piggy- backs on a conventional colonoscope. During a colonoscopy, a clinician can press the fiber-optic probe with the 2-mm-diameter microobjective against suspicious tissue and visualize vascular and cellular changes that may indicate cancer. Since the imaging is performed in real time, clinicians can make immediate decisions about whether further treatment is needed.
With HiLo microendoscopy, “we may be able to limit the number of biopsies patients undergo and reduce the risks associated with those biopsies such as bleeding and infection,” says Ford. The technique also may reduce costs for diagnosis since fewer pathology lab services may be needed.
Quantum dots are key for Raman/fluorescence combo
Raman microscopy captures chemical data from single cells providing information such as the concentration of biomolecules and details about the local environment. Fluorescence imaging allows researchers to pinpoint single molecules in a sample. However, researchers normally don’t combine the two techniques. The much stronger fluorescence signals can overwhelm subtle Raman signals. But Cees Otto and his colleagues at the University of Twente (Enschede, The Netherlands) believe merging the two techniques will create a powerful imaging tool to reveal important information about cell activities.
The key to the confocal Raman/fluorescence combo is the use of quantum dots. These bright, stable, tiny spheres can be tailored to achieve desirable optical properties. “If you choose the right quantum dot, the light emitted won’t overlap with Raman signals,” explains Henk-Jan van Manen, who as a postdoctoral student collaborated with Otto on the technique.
In an early experiment, Otto and van Manen mixed quantum dots with cells and then irradiated the mixture with continuous-wave laser light at 413 nm. The Raman signal from cells occurred between 420 and 445 nm. One-photon excitation fluorescence of the quantum dots was detected between 590 and 650 nm (see Fig. 2). When the wavelength increased to 647 nm, non-resonant Raman signals occurred between 660 and 730 nm and two-photon excitation occurred between 560 and 640 nm (see Fig. 3).
After van Manen and Otto established the proof-of-concept, they applied the technique to imaging specific structures inside a cell. Using the quantum dots saves time because “the quantum dots are like a flashlight,” says van Manen, now at AkzoNobel, headquartered in The Netherlands. “They point to an interesting location and we are then able to get detailed information using Raman imaging.” Label-free spontaneous Raman spectroscopy provides cell information quickly and has a better signal-to-noise ratio than coherent anti-Stokes Raman spectroscopy.
Actual imaging times have dropped significantly since 2003, when Otto began investigating the union of Raman and fluorescence microscopy. Eight years ago image acquisition time was about 17 minutes for a 32 × 32 image, roughly 1 sec/pixel. Today, the hybrid confocal Raman fluorescence microscopy approach imaging rates hover at two minutes for a 32 × 32 image, roughly 0.1 sec/pixel.
Improved light sources, detectors, hard coatings on optical components, and microscope objectives all contribute to the technique’s faster speed, says Otto. He says that the rate limiting step now is computer power: “We can take an image faster than the computer can process it.”
Faster imaging times will allow Otto and his team to explore a host of cellular activities and to take full advantage of Raman spectroscopy’s label-free approach. Recently they examined the ratio of RNA and DNA at the edge of nuclei, demonstrating that RNA is in greater abundance than DNA. Otto is now investigating the use of the hybrid technique to provide Raman signatures for a variety of cells. Beyond research applications, Otto and van Manen anticipate uses in the pharmaceutical industry to determine formulations and drug efficacy and in clinical diagnostics.
Imaging living cells and tissue requires an elegant balance of illumination, detection, and processing. Hybrid techniques will allow researchers to extract important quantitative data from their images and use that information to deepen our understanding of fundamental biological processes, disease states, and other critical activities that occur on microscopic scales.
References
1. H.J. van Manen and C. Otto, Nano Lett. 7, 6, 1631–1636 (2007).
2. D. Lim et al., J. Biomed. Opt. 16, 1 (2011).
More BioOptics World Current Issue Articles
More BioOptics World Archives Issue Articles
About the Author
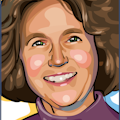