Optical stimulation connects electrical and biological neurons
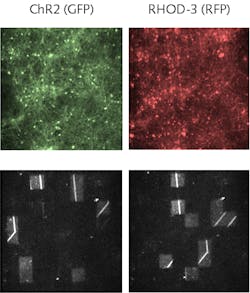
Individual neurons detect and initiate electrochemical signals. When they group together, the simple action of a single neuron leads to complex behaviors across a variety of spatial and temporal scales. Unfortunately, when communication between neurons is disrupted, complex behavior is disrupted as well. The Brain Bow Project, a Future and Emerging technologies program of the European Union that ended in 2015, was designed to investigate the mechanisms of neural communication. A cross-disciplinary international team of researchers who initially collaborated on Brain Bow continue to investigate the workings of neural signaling.
Professor Timothée Levi of the University of Bordeaux (France) and his colleagues at the University of Tel Aviv (Israel) and IkerBasque Biocruces (Bilbao, Spain) have developed a proof-of-principle communication system that transmits signals from an electronic neural circuit simulator to living neural tissue via patterned optical illumination.1 The electronic signal is generated in a spiking neural network (SNN), patterned by a digital light projector, and then used to stimulate a neural tissue culture—a biological neural network (BNN).
Linking electrical signals to neural responses
The communication starts as a signal generated at the SNN, implemented in a field-programmable gate array (FPGA) configured to emulate a biological neural network. The SNN is composed of 100 “Izhikevich neurons”—a straightforward mathematical simulation that simplifies high-fidelity modeling of biological neurons.
The Izhikevich model can represent different types of neural activity, simply by changing a few parameters. In this implementation, the researchers created an SNN with 80 excitatory and 20 inhibitory neurons. As the SNN runs, it emulates a biological network, including duplicating features such as spontaneous network synchronizations, where neurons influence each other to fire simultaneously. For different experiments, the neuron parameters can be tweaked to present different realistic scenarios, including the effects of plasticity, axonal delay, and synaptic noise.
The FPGA architecture allows for real-time, low-power implementation, and also incorporates additional functionality. The researchers defined a subgroup of 64 neurons, an 8 × 8 array of “on” or “off” depending upon the firing state of the associated neuron. Another stage of the FPGA converted that to a VGA signal to drive the central 600 × 600 pixels of an 800 × 600-pixel digital projector in which the light source had been replaced with a high-power blue LED. Relay optics project the image of the video projector’s digital micromirror device (DMD) into a microscope, creating a “checkerboard” of blue squares at the object plane. The biological neural network is placed at that object plane.
The BNN was fabricated by culturing embryonic mouse cortical cells, during which they were transfected with ChIEF, a genetic modification that allows neural activity to be optically stimulated at physiologically relevant frequencies. The cells were cultured on a multielectrode array (MEA) with a cell density of 3000 to 4000 cells per square millimeter. The cells were incubated with the calcium sensor RHOD-3, which emits red fluorescence when bound to calcium. With appropriate optical filters, a mercury lamp provides the excitation light; an EMCCD acquires the calcium images (see figure). These images, combined with electrical measurements provided by the MEA, record the BNN’s response to the light pattern.
The researchers defined a series of experiments with different SNN parameters. In a typical experiment, they recorded the BNN activity for about 50 seconds and then turned on the optical communication. The neurons of the SNN emulate a biological network, with the state of the 8 × 8 neural array changing at a selectable rate. When the number of excited neurons exceeds a defined threshold, the LED light is turned on, and the BNN is illuminated with the checkerboard pattern corresponding to the 8 × 8 SNN neurons. Simultaneously, BNN activity is continuously recorded, allowing the researchers to quantify the degree of correlation between the SNN stimulus and BNN response.
Quantifying interconnection
In a neural network, when a given number of neurons spike, they trigger collective behavior. If communication between two halves of the network is severed, spiking in one portion of the network cannot influence neurons in the other half. The SNN/BNN combination reflects this behavior. Before the optical interconnect is turned on, the SNN and BNN are unsynchronized, but with the interconnect engaged, signals originating in the SNN entrain signals in the BNN. Specifically, if there is a high degree of pattern similarity in the SNN, it produces a comparable degree of similarity in the BNN.
“This technique offers much more potential than classical electrical stimuli,” says Levi, “with higher spatial resolution and the possibility to optogenetically modify selective neuronal subpopulations.” With the basic functionality demonstrated, he says, “we plan to implement a single-neuron to single-neuron only-optical closed-loop in vivo animal system, to see, for example, if the SNN is able to detect and trigger epileptic events.”
REFERENCE
1. Y. Mosbacher et al., Sci. Rep., 10, 7512 (2020); doi:10.1038/s41598-020-63934-4.
About the Author
Richard Gaughan
Contributing Writer, BioOptics World
Richard Gaughan is the Owner of Mountain Optical Systems and a contributing writer for BioOptics World.