Neuroscience/Optogenetics: All-optical, high-precision functional optogenetics
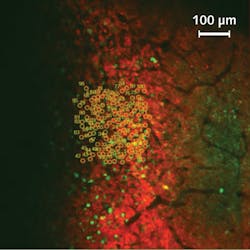
Understanding the brain's ongoing processes at the cellular level not only promises to help determine the physiological basis of cognition, but also nurtures the hope of curing brain diseases that are currently difficult or impossible to treat. Achieving this understanding—that is, determining the brain's functional network structure and how that structure links to cognition and behavior—requires the ability to record the activity of large numbers of neurons, and to manipulate and probe that activity.
In groundbreaking experiments, neuroscientists from the group of Professor Michael Häusser at University College London have succeeded in observing and controlling the activity of defined cell types at an unprecedented level by way of optogenetics. The work leverages important new developments in light-sensitive probes and genetically encoded activity sensors, plus novel optical hardware, that together enable recording and manipulation of neural circuit activity—with single-neuron and single-action potential precision—using only light in the intact brain.
Deep-tissue imaging and photostimulation
Although the basic mechanism of neural activity is well understood, the complex interconnection of specific neurons or groups of neurons, and the behavioral pattern they are linked to, are not—and thus require novel and interdisciplinary techniques of investigation. Häusser's team has managed to observe the activity of >1000 single neurons simultaneously, while manipulating the activity of 100 targeted neurons to determine the extent to which their action is reproducibly linked to a behavior.
Neurons communicate with one another through electric potentials that migrate as signals along the cell membrane. The level of positively and negatively charged ions present in and around the cells control their activity. When an action potential is triggered, calcium (Ca2+) ions flow through channels in the membrane into the neuron.
Häusser and his team genetically modified the brain of a mouse by inserting two different proteins: a calcium-sensitive fluorophore and a light-sensitive ion channel. The ion channel inserted into the cell membrane can be optically triggered to cause sodium (Na+) ions to flow into the cell, resulting in a neural action potential. The action potential then opens voltage-gated Ca2+ channels, and Ca2+ ions flow into the cell and increase the fluorescence. Because the fluorescence is easily visible, it is easy to confirm success of the stimulation—and thus neural activity.The pattern is achieved by repeatedly applying a spiral motion of the stimulating laser focus over the area of interest. Within this pattern, the field illuminated by the stimulating laser includes only neurons that have been genetically modified and identified (through observation of their behavioral correlates—for instance, firing in response to a specific sensory stimulus) as potentially guiding behavior. In this way, the neural computation can be causally probed within a brain region, and specific activity patterns of certain neurons can be linked to the behavioral output of the animal. The stimulating laser power, duration, and temporal pattern can be set to drive very well-defined activity patterns in the targeted neurons.
In vivo photon triggering of action potentials
With the novel photostimulation and imaging setup, the UCL researchers are pioneering in the field of targeted optogenetics. They have selectively activated 100 neurons in the brain of an awake and behaving mouse (see https://youtu.be/dhT8-Ri06wQ). The animal's running activity is coupled to movement around a virtual reality environment, allowing it to perform complex spatial tasks while being head-fixed to enable effective and accurate imaging and stimulation.
The current example uses 1040 nm femtosecond pulses-at an average power of 6 mW per cell and 100 ms exposure duration-to trigger neural activity. Since the photoactivation process is based on multiphoton absorption of the near-infrared wavelength laser, the resulting high spatial resolution ensures the ability to address selected neurons without affecting neighboring cells.
The video-recorded experiment shows the average fluorescence response of the calcium sensitive protein to 10 stimulation cycles. To isolate the effect of the stimulus on the cell activity, the change in fluorescence (∆F) is divided by the fluorescence (F). Figure 4 captures the average ∆F/F 0.3 s after photostimulation, and depicts the temporal progress of the fluorescence increase ∆F/F in each cell resulting from the stimulating laser pulse. On average, the signal increases by more than 130%.The all-optical technique being developed by Prof. Häusser's group promises to enable great progress in neuroscientific research. It allows targeting of neurons based not just on anatomy or genetic properties, but on functional attributes. It also allows the recording and manipulation of neural circuits at both spatial and temporal resolution that correlates with normal operation as the subject moves. Thus, it offers a new approach to understanding how activity in defined groups of neurons have a causative effect on behavior.
Michael Mei | CEO, Menlo Systems
Michael Mei is CEO at Menlo Systems (Martinsried, Germany).
Nick Robinson | Research Associate, Wolfson Institute for Biomedical Research
Nick Robinson is a research associate at the Wolfson Institute for Biomedical Research, Division of Medicine, at University College London (UK).