RAMAN SPECTROSCOPY: Micro-Raman spectra explore carbon nanotube geometry
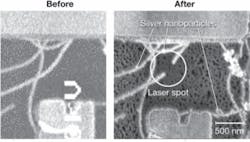
Raman spectroscopy enables chemical identification of many substances through the vibrational energies of molecules, but the scattering cross section of most molecules is extremely small, limiting its use. A technique called surface-enhanced Raman spectroscopy (SERS) improves the small Raman intensities, making related applications more practical1 (see www.laserfocusworld.com/articles/274732). Researchers at the University of Southern California (USC), Los Angeles, led by assistant professor of electrical engineering Stephen Cronin, conducted a systematic study of the interaction between SERS and individual carbon nanotubes to explore the SERS enhancement effect and the geometry of nanotubes.
Previous efforts using SERS on solutions and metal surfaces haven’t offered much information about the geometry of single nanoparticles or molecules. The use of SERS on carbon nanotubes takes advantage of the nanotubes’ length and conducting properties to attempt to measure properties like the distance between nanoparticles and Raman active molecules, the number of molecules on each nanoparticle, and the extent of plasmonic coupling between nanoparticles.
“The uniquely large aspect ratio of carbon nanotubes enables imaging of the nanoparticle geometry together with the SERS active molecule,” says Ronin.
The group used chemical vapor deposition to grow carbon nanotubes on a silicon/silicon dioxide substrate. A 6-nm-thick film of silver (Ag) was applied to form nanometer-size islands on the sample, to serve as SERS-enhancing nanoparticles. A micro-Raman spectrometer with a 0.5-μm-diameter spot size collected spectra of approximately 100 nanotubes before and after depositing the silver. Exposing the nanotubes to 2 mW of 633 nm laser light for 120 s before the silver nanoparticle deposition resulted an intensity of 58 photon counts. After deposition, the same laser exposure at the same location showed an intensity of 19,450 photon counts, an enhancement factor of 335 times. The enhancement is due to the surface-plasmon resonance of the Ag nanoparticles, say the researchers, which couples the laser light very efficiently into the nanotube. Because the SERS-active region had an effective area of 25 nm, 400 times smaller than the laser spot size, the team estimates the true enhancement factor was 400 times that indicated by the spectra, or 134,000.
Several nanotubes exhibited SERS-induced burnout, caused by the oscillation of the plasmonic charge on the surface of the Ag nanoparticles (see figure). Prior research found that carbon nanotubes burn out at 600°C in air, so the experiment demonstrates that laser powers of 20 mW can induce nanoparticles to reach temperatures exceeding 600°C. Although a large region containing several nanotubes was exposed to the 20 mW laser light, only the SERS-active region exhibited burnout, demonstrating the spatial rarity of such an event.
Because the silver nanoparticles are distributed randomly, the researchers estimate that the occurrence of SERS-induced hot spots is very rare indeed. In future work, careful spacing of the Ag nanoparticles during deposition will increase the chances of this rare event. “More effective techniques will provide higher densities of SERS hot spots and, hence, higher overall Raman signals,” says Ronin. “This will make new applications in chemical and biochemical sensing and identification feasible with handheld spectrometers, which typically have low quantum efficiencies and spectral resolution.”
REFERENCE
1. R. Kumar et al., Appl. Phys. Lett.91, 223105, (Nov. 26, 2007).
About the Author
Valerie Coffey-Rosich
Contributing Editor
Valerie Coffey-Rosich is a freelance science and technology writer and editor and a contributing editor for Laser Focus World; she previously served as an Associate Technical Editor (2000-2003) and a Senior Technical Editor (2007-2008) for Laser Focus World.
Valerie holds a BS in physics from the University of Nevada, Reno, and an MA in astronomy from Boston University. She specializes in editing and writing about optics, photonics, astronomy, and physics in academic, reference, and business-to-business publications. In addition to Laser Focus World, her work has appeared online and in print for clients such as the American Institute of Physics, American Heritage Dictionary, BioPhotonics, Encyclopedia Britannica, EuroPhotonics, the Optical Society of America, Photonics Focus, Photonics Spectra, Sky & Telescope, and many others. She is based in Palm Springs, California.