Fiber Lasers: Ultranarrow Brillouin fiber laser has built-in temperature stability
To achieve ultranarrow laser linewidths of 1 Hz or less for applications in precision spectroscopy and metrology, the typical ultralow-expansion (ULE) cavity-stabilized laser meets the linewidth requirements, but falls short of amenability to portable applications due to its large size (a cubic meter or so) and vibration susceptibility. Combining the inherent robustness of a fiber-laser architecture and stimulated Brillouin scattering (SBS) in a high-quality (Q) resonator, Massachusetts Institute of Technology (MIT) Lincoln Laboratory (Lexington, MA) researchers have achieved ultranarrow linewidth lasing in a portable package that provides built-in temperature stability.1
High-Q temperature stability
The high-Q, temperature-stabilized laser cavity consists of two independent, orthogonally polarized pump laser signals sent into a single resonator, generating two orthogonal polarization SBS signals. Components in the setup include phase modulators and semiconductor optical amplifiers (SOAs) to boost power to around 15 mW (see figure). These two signals then travel backward through the circulator and their frequency beat becomes the temperature-correction signal.
Pump light is monitored and stabilized to the cavity resonance, and the optical-fiber resonator is secured to a block of aluminum placed on vibration-dampening foam in order to keep the fibers secure and isolate the system from table vibrations.
To control the coupling ratio of the resonator to generate ultranarrow-linewidth lasing, the transverse offset between two polished fibers in the resonator cavity is controlled. The narrowest lasing linewidth occurs for a coupling ratio of around 1.3%, but the researchers used 5.6% coupling to maximize output power without degrading the laser linewidth (which is around 20 Hz full-width half-maximum for a fairly wide range of coupling ratios). The long lengths of fiber and large mode-field diameter fundamentally increase the optical mode volume.
Dramatic reduction in the long-term frequency drift of the SBS laser (around 1.65 GHz for a 1°C temperature drift in silica glass) is achieved through the polarization-based temperature-sensing technique wherein the change in frequency of the beat signal described previously corresponds to a direct temperature change in the optical resonator. Temperature stabilization is accomplished via a resistive heater—that is, heat is applied to the optical fiber by increasing or decreasing the applied current to the resistive heater in order to keep the SBS beat frequency constant.
The temperature-stabilized SBS laser affords a significant improvement in the rejection of temperature disturbances compared to standard SBS lasers and allows for shifts as small as 85 nK to be corrected. Furthermore, many of the components in the setup could be integrated in an on-chip format for compact assembly and portable applications.
“Many applications in basic and applied science, such as optical atomic clocks, gravitational wave detection, and advanced lidar, fundamentally rely on lasers of extreme frequency precision for their operation,” says MIT Lincoln Laboratory staff scientist William Loh. “In order to eventually transition these systems into the field or space, one critical step involves miniaturizing the stable laser source without degrading its precision. By advancing this SBS laser technology, we aim to bridge the wide gap in performance between the scientific-grade but otherwise bulky ULE cavity-stabilized lasers and the rest of the laser technologies in existence today.”
REFERENCE
1. W. Loh et al., Optica, 6, 2, 152–159 (2019).
About the Author
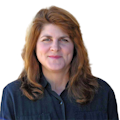
Gail Overton
Senior Editor (2004-2020)
Gail has more than 30 years of engineering, marketing, product management, and editorial experience in the photonics and optical communications industry. Before joining the staff at Laser Focus World in 2004, she held many product management and product marketing roles in the fiber-optics industry, most notably at Hughes (El Segundo, CA), GTE Labs (Waltham, MA), Corning (Corning, NY), Photon Kinetics (Beaverton, OR), and Newport Corporation (Irvine, CA). During her marketing career, Gail published articles in WDM Solutions and Sensors magazine and traveled internationally to conduct product and sales training. Gail received her BS degree in physics, with an emphasis in optics, from San Diego State University in San Diego, CA in May 1986.