Laser Microfabrication: Laser-written flexible waveguide in polymer could spawn new medical devices
Writing optical waveguides in transparent optical materials is a decades-old technique, at least for rigid materials like glass and polymers. In addition, optical waveguides have been written in polymers using electron beams and gamma rays. Now, researchers at the École Polytechnique Fédérale de Lausanne (EPFL; Lausanne, Switzerland) have for the first time fabricated flexible waveguides about 1.3 µm wide in polydimethylsiloxane (PDMS), a clear silicone commonly used for biomedical applications. The waveguides can be used to make light-based devices such as biomedical sensors and endoscopes that are smaller and more complex than currently possible.
The optical waveguides have a transmission loss of only 0.03 dB/cm in the 650–700 nm spectral range, meaning that a light-based signal can travel through the new waveguides for 10 cm or more before an unacceptable degradation of the signal occurs.
The researchers made the new waveguides by optimizing laser direct writing, a microfabrication approach that creates detailed 3D structures by polymerizing a light-sensitive chemical with a precisely positioned focused laser. Polymerization converts relatively small molecules called monomers into large, chainlike polymers. They used femtosecond titanium sapphire (Ti:sapphire) laser tuned to a 680 nm central wavelength and emitting pulses at an 80 MHz repetition rate and a 140 fs duration. Writing speeds were between 0.5 and 1.5 mm/s—using a microscope objective with a 0.7 numerical aperture (NA), the researchers estimate that the peak intensity at the lens focus was about 2 × 1012 W/cm2.
The new approach does not require a photoinitiator, which is typically used to efficiently absorb the laser light and convert it into chemical energy that initiates polymerization. “By not using a photoinitiator, we simplified the fabrication process and also enhanced the compatibility of the final device with living tissue,” says research team member Ye Pu. “This enhanced biocompatibility could allow the approach to be used to make implantable sensors and devices.”
To achieve a small optical waveguide that efficiently confines light, there must be a large difference between the refractive index of the material making up the waveguides and the surrounding PDMS. The researchers loaded phenylacetylene into the PDMS for the waveguides (by soaking the PDMS in liquid phenylacetylene) because, compared to traditionally used materials, phenylacetylene has a higher refractive index once polymerized.
Multiphoton absorption
The focused ultrafast laser pulses induced multiphoton absorption in the material. Multiphoton laser direct writing produces much finer structures than one-photon processes because the volume of polymerization at each writing spot is much smaller. Using multiphoton laser direct writing also allowed the researchers to directly initiate phenylacetylene polymerization without a photoinitiator. They then evaporated any unpolymerized phenylacetylene by heating the PDMS.
“To the best of our knowledge, these are the smallest optical waveguides ever created in polydimethylsiloxane, or PDMS,” Pu says. “Our flexible waveguides could be integrated into microfluidic lab-on-a-chip systems to eliminate bulky external optics needed to perform blood tests, for example. They might also deliver light for wearable devices such as a shirt featuring a display.”
The new flexible waveguides could also serve as building blocks for photonic printed-circuit boards.
The researchers are now working to improve the yield of the fabrication process by developing a control system that would help avoid material damage during laser writing. They also plan to create an array of narrow waveguides in PDMS that could be used to construct a very flexible endoscope with a diameter <1 mm.
“Such a small, mechanically flexible endoscope would allow a number of hard-to-reach places in the body to be imaged for diagnosis in the clinic, or for monitoring in a minimally invasive surgery,” Pu says.
REFERENCE
1. G. Panusa et al., Opt. Mater. Express (2018); https://doi.org/10.1364/ome.9.000128.
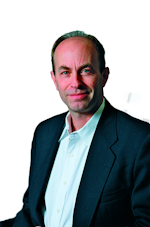
John Wallace | Senior Technical Editor (1998-2022)
John Wallace was with Laser Focus World for nearly 25 years, retiring in late June 2022. He obtained a bachelor's degree in mechanical engineering and physics at Rutgers University and a master's in optical engineering at the University of Rochester. Before becoming an editor, John worked as an engineer at RCA, Exxon, Eastman Kodak, and GCA Corporation.