Lattice-trapped atoms are a precise time reference
The existing definition of the second is based on a microwave transition between two hyperfine levels of cesium (9,192,631,770 periods at 0 K). As a result of the development of an optical-frequency-comb technique that establishes a coherent link between optical and radio frequencies, optical clocks are increasingly being studied as future atomic clocks with superior precision. Ultracold neutral atoms in free fall and single trapped ions have shown performance close to that of cesium clocks.
Now, researchers at the University of Tokyo (Tokyo), the Japan Science and Technology Agency (Tokyo, Japan), and the National Metrology Institute of Japan (Ibaraki) have developed a so-called optical lattice clock that is based on strontium (Sr) atoms trapped in an optical lattice formed by the spatial-interference pattern of lasers as quantum references. This optical clock has a linewidth one order of magnitude narrower than neutral-atom clocks, and better stability than single-ion clocks.
Optical clocks have high stability because they use atomic transitions at frequencies higher than microwaves. This higher frequency, coupled with the use of a well-designed atom trap that increases coherent interaction time and reduces the Fourier linewidth, can result in extremely accurate optical clocks. If a single quantum absorber can be held in a space smaller than the transition wavelength, then atomic interactions and Doppler shifts that affect clock accuracy can be diminished.
To demonstrate this optical-lattice clock, the researchers laser-cooled Sr atoms on the 1S0 to 3P1 transition and trapped them using a one-dimensional optical lattice formed by the standing wave of a Ti:sapphire laser at 813.4 nm. Approximately 104 atoms at a temperature of about 2 µK were loaded into the 20-µK-deep lattice and were irradiated by the clock laser (serving as a reference cavity) operating at 698 nm for 10 to 40 ms to excite atoms trapped in the optical lattice to the 3P0 state (see figure). The excitation of the clock transition was then monitored by laser-induced fluorescence on the 1S0 to 1P1 cyclic transition. The researchers observed a Fourier-limited linewidth of 27 Hz, the narrowest linewidth reported for a neutral-atom-based optical clock. Using a frequency comb to measure the clock laser against a commercial cesium clock, an uncertainty of 15 Hz was recorded for the optical-lattice clock.
The key to the improved accuracy and stability of the lattice clock is the ability to trap atoms using the optical lattice operating at a “magic” wavelength. Usually, a lattice-trapping field modifies the internal states of atoms by so-called light shifts, which degrade the accuracy of optical clocks. However, a light-shift-cancellation technique was developed whereby the atomic transition frequency is equal to the unperturbed transition frequency independent of the lattice laser intensity-in effect, the light shifts are eliminated at this “magic” lattice-laser wavelength and the most stable transition is observed.
The researchers are exploring lattice clocks that would operate with different atomic species than the 87Sr used in this experiment. “A frequency comparison of these different lattice clocks would allow us to test the time variation of physical constants,” says researcher Hidetoshi Katori. “We expect that this optical-lattice clock would allow measuring fractional frequency differences on the 10-18 level in one second of measurement time.”
REFERENCE
1. M. Takamoto et al., Nature 435 (May 19, 2005).
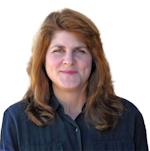
Gail Overton | Senior Editor (2004-2020)
Gail has more than 30 years of engineering, marketing, product management, and editorial experience in the photonics and optical communications industry. Before joining the staff at Laser Focus World in 2004, she held many product management and product marketing roles in the fiber-optics industry, most notably at Hughes (El Segundo, CA), GTE Labs (Waltham, MA), Corning (Corning, NY), Photon Kinetics (Beaverton, OR), and Newport Corporation (Irvine, CA). During her marketing career, Gail published articles in WDM Solutions and Sensors magazine and traveled internationally to conduct product and sales training. Gail received her BS degree in physics, with an emphasis in optics, from San Diego State University in San Diego, CA in May 1986.