Surface plasmons boost emission from dyed polymer
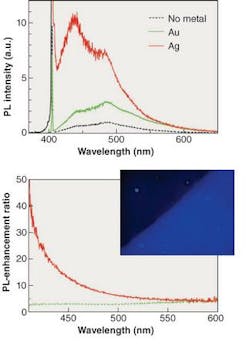
The same phenomenon that allows metal nanoparticles to enhance fluorescence of dyes for biomedical applications (see Laser Focus World, June 2005, p. 47) has been applied by researchers at the California Institute of Technology (Caltech; Pasadena, CA) to boost the output of polymer dyes, which are useful for organic light-emitting diodes (OLEDs). In both cases, surface-plasmon resonance is the key.1
When light of the right wavelength hits certain metals, defects or structures on the metal’s surface can couple the light’s energy into electron oscillations called surface plasmons. The same defects or structures can convert the plasmons back into light. Resonances can occur, with a strength and spectral range depending on the metal. Gold, silver, and aluminum are all candidate metals for exploiting this effect.
In the Caltech experiment, one-half of a quartz substrate was first coated with a metal film and then with a dye-doped polymer-Coumarin 460 laser dye in polymethylmethacrylate (PMMA)-to form the test sample (Coumarin 460 emits at 460 nm upon UV excitation). The other half was coated only with the dyed polymer to form the control sample. Metal films 50 nm thick of gold or silver were used; the polymer coating was 200‑nm thick-much thinner than the dye’s excitation-penetration depth of 646 nm at the experimental concentration.
The UV pump light was introduced at a large angle to the normal, while the detector was placed directly above the sample to minimize pump-light collection by the detector. The presence of a gold film boosted the output of the dye by approximately a factor of two evenly across the emission spectrum of the dye; this was attributed largely to reflection from the gold.
The silver, however, was a different story. The dielectric constants of silver combined with the properties of PMMA predict a plasmon resonance that ties in with Coumarin 460’s emission, and, indeed, the researchers observed an elevenfold enhancement in emission at 460 nm (see figure). In fact, at wavelengths shorter than the dye’s peak, enhancement ratios of up to 50 were seen. The exchange of energy between pump photons and surface plasmons occurred primarily through flaws and roughness in the silver surface.
The higher photoluminescence-enhancement ratio at shorter wavelengths has caught the researchers attention; they plan to take advantage of shorter-wavelength (UV) dyes by using other metals (for example, aluminum) as well as experiment with other dyes at shorter wavelengths in combination with silver, says Terrell Neal, one of the researchers.
Patterned metal layers can further raise the efficiency of the enhancement effect, note the researchers. Such “geometric tuning” can be accomplished with photolithographic techniques and would result in gratings, dot arrays, or other periodic structures. “We have to design the pattern to tune the surface-plasmon frequency to match to the emission wavelength and to extract the light more effectively,” Neal says. The Caltech researchers aim to fabricate an electrically pumped plasmon-enhanced OLED.
REFERENCE
1. T. D. Neal et al., Optics Express13, 14 (July 11, 2005).
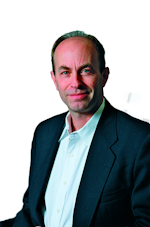
John Wallace | Senior Technical Editor (1998-2022)
John Wallace was with Laser Focus World for nearly 25 years, retiring in late June 2022. He obtained a bachelor's degree in mechanical engineering and physics at Rutgers University and a master's in optical engineering at the University of Rochester. Before becoming an editor, John worked as an engineer at RCA, Exxon, Eastman Kodak, and GCA Corporation.