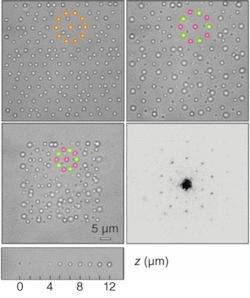
The higher the degree of symmetry a 2-D photonic crystal (PC) has, the better its properties tend to be. As the symmetry goes up, the required refractive-index contrast to achieve a complete photonic bandgap tends to diminish; in addition, optical properties grow more isotropic (less sensitive to angle). While ordinary 2-D PCs can have up to sixfold (hexagonal) rotational symmetry, a class of 2-D photonic structures called quasicrystals can have much higher degrees of symmetry-twelvefold and more-and can be made into practical devices (see Laser Focus World, July 2000, p. 17, and April 2005, p. 13). While not strictly crystalline, these structures have long-range order.
Now, Yael Roichman and David Grier of New York University (NYU; New York, NY) have brought quasicrystallinity to 3-D photonic structures.1 While 3‑D PCs have been fabricated in many ways, including colloidal self-assembly and photolithography, existing 3-D photonic structures all have symmetries similar to those found in common crystals. Instead, the NYU researchers have created quasicrystalline photonic structures that use the icosahedron (a 3-D shape that has 20 equilateral triangles as sides) as the basic building block-creating the potential for 3-D structures with easier-to-achieve photonic bandgaps.
Because of the complicated geometry and the researchers’ desire to create a high-quality structure that they could replicate without error, they settled on an assembly method based on optical trapping. In the technique, colloidal silica microspheres are caught and moved into place within a clear UV-curing gel. Computer-generated holograms projected through an oil-immersion microscope objective (numerical aperture of 1.4) create large arrays of optical traps that allow precise positioning of each and every element in the structure at once.
The 1.53-µm microspheres are heavy and at first settle into a monolayer on the glass coverslip. The researchers trap them, forming them into a 2-D quasicrystal, and then adjust their axial positions to form a 3-D quasicrystal (see figure). Ultraviolet curing fixes the array.
“We move our traps from one configuration to another by projecting a sequence of holograms, in close analogy with a conventional cartoon animation,” says Grier. “The difference is that cartoons animate light with matter, and we’re animating matter with light. The holograms are encoded on a liquid-crystal spatial-light modulator, whose phase pattern is updated with a computer. So there are no moving parts (other than the liquid-crystal molecules).”
Scaling up
Using the existing technology, which is not optimized for manufacturing, the researchers can make a single 3-D domain with just about any structure (including quasicrystalline) consisting of several hundred spheres. The number of elements can be scaled up in three ways, Grier notes. First, the fabrication method can be optimized by using wide-field objective lenses for projection, higher-power lasers, and larger and faster spatial-light modulators. Second, larger structures can be built hierarchically by combining holographically assembled domains. Finally, holographically organized structures can be built up into larger arrays through a repetitive shoot-and-step process. “These extensions should enable the fabrication of arbitrarily large and complex 3-D structures with micrometer-scale features,” says Grier.
At the moment, the most time-consuming part of the process is fishing for the particles, Grier notes. “This could be overcome quite easily by integrating microfluidic sample handling to deliver spheres to the traps. Once enough spheres are trapped in a convenient starting configuration, 3-D assembly would proceed without intervention in a matter of seconds. It also could be made much faster with hardware optimization.”
The holographic-assembly method can potentially be exploited to embed gain media in photonic-bandgap cavities and position nonlinear materials in waveguides to form photonic components such as optical switches. “There’s a whole panoply of 2-D and 3-D quasicrystalline structures,” notes Grier. “It’s not clear that all have been discovered yet.”
REFERENCE
1. Y. Roichman and D. G. Grier, Optics Express, 13(14) (July 11, 2005).
About the Author
John Wallace
Senior Technical Editor (1998-2022)
John Wallace was with Laser Focus World for nearly 25 years, retiring in late June 2022. He obtained a bachelor's degree in mechanical engineering and physics at Rutgers University and a master's in optical engineering at the University of Rochester. Before becoming an editor, John worked as an engineer at RCA, Exxon, Eastman Kodak, and GCA Corporation.