ADVANCED APPLICATIONS: HOLOGRAPHY: Holographic data storage chips away at barriers
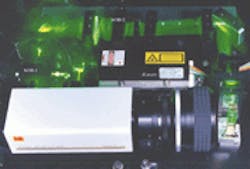
For decades, holographic optical data storage has been losing in a race with silicon-based memories and magnetic and optical disks. As researchers have developed ways to make holographic methods more practical and data storage denser, conventional techniques have progressed faster, pushing the technical hurdles to commercialization ever higher. However, accelerating progress in multiple-frequency holography, based on spectral hole burning, offers at least the theoretical and perhaps practical potential for leapfrogging to such high data densities and readout speeds that the holographic turtle may still win the race. Practical prototypes of more modest systems also have shown that holographic data storage does not have to be trapped in the laboratory forever.
Holograms vs. DRAMs and disks
In holographic data storage, data are first turned into a two-dimensional pattern of light by a spatial light modulator (SLM; an array of light switches that can store as many as one million bits or pixels). Laser light is beamed through the SLM to the recording medium, such as a photopolymer, while a reference beam also illuminates the medium so that interference patterns are created. These expose the medium by generating corresponding differences in optical properties such as refractive index or absorption. Many pages of holograms can be multiplexed onto the same medium, either by varying the angle or phase of the reference beam, or by using different frequencies, among other techniques.
The extremely high data densities that, in theory, can be obtained by holography have made it an attractive alternative to DRAMs or optical data disks. Using spectral hole burning, as many as 105 bits can be recorded in a single 1-µm cube, allowing surface densities of more than 1 million bits/µm2 with relatively thin holographic coatings. By comparison, current DRAMs have storage densities of about 1 bit/µm2, while optical and magnetic disks are at about 8 bits/µm2. In addition, because holography reads out and records entire pages of data at a time, read/write rates of 1 Tbit/s can, in theory, be obtained.
Holographic systems are far from commercial reality, however, so the real comparison is not with current systems but with those that will exist in six or seven years—about the minimum time for commercialization of holographic data storage. The comparison with DRAMs then becomes far less optimistic for holography, because, by 2006, DRAMs are expected to achieve read/write rates of more than 2 Tbits/s, with data densities of only about 25 bits/µm2. But the comparison with optical disks is still likely to be favorable, because disk read/write speeds—limited by the speed a disk can spin—are expected to be only around 100 Mbit/s in 2006. The goal of replacing next-generation read-only recording media with holographic storage is, therefore, a tempting target for researchers.
Burning holes in the spectrum
Much of the current focus of holographic-data-storage research is development of spectral hole-burning techniques that allow many bits to be stored in a single location with the dimensions of a single wavelength. For this to work, the absorption band of the recording medium must consist of a large number of very narrow-bandwidth individual absorbers. When the material is excited with narrow-bandwidth laser light, only those absorbers in resonance with the radiation will transition to an excited electronic state. If there is an alternative state that the excited absorbers can decay to (other than the ground state), then the absorbers will no longer be in resonance with the exciting radiation. As a result, a hole is burned in the absorption spectrum because there are fewer absorbers available.
Clearly, this technique requires that the alternative state be relatively stable so that the information stored is retained. In most cases, this requires cooling the materials to very low temperatures. In addition, a second, or gating, frequency is sometimes used to boost the excited absorbers into a more stable state (see Fig. 1). This also means that exposure, during the read process, to the original exciting radiation will not affect the number of excited states, so that reading will not be a destructive process.The number of bits that can be stored in a given region of material equal in size to a wavelength of light is given by the ratio of the inhomogeneous linewidth (that of the entire absorbing band) to the homogenous linewidth (the width of individual narrow-bandwidth absorbers). In some materials this can be as high as 108 but is often between 103 and 105, giving the potential for high data-storage densities.1
In spatial-spectral holography, two pulsed beams, each within the broad (inhomogeneous) bandwidth of the material and each shorter in duration that the lifetime of the narrow-bandwidth (homogenous) transition, illuminate the medium with a slight temporal spacing and different angles. This produces an inference pattern in both space and frequency, a spatial-spectral hologram.
Not only can data be stored holographically in this manner, but the information can be processed as well. When two pulses in sequence are used to hole-burn, the resulting pattern is given by multiplication of the bit stream included in each. Again, in theory, multiplication rates of terabits per second can be performed at each spatial location, which may be only 1 µm across.
Materials are key
Translating these theoretical promises into practical reality rests on developing the appropriate materials to act as holographic media. It is here that some real progress is being made, but the challenges are formidable.
First, lattice vibrations smear out the narrow line resonances, so devices have to be kept at low temperatures. At such low temperatures—around the boiling point for helium (4 K)—several organic dye molecules exhibit spectrum hole burning with subnanosecond hole-burning times and terahertz-wide inhomogeneous bandwidths. But using the gated burning techniques needed to avoid erasure during reading sends burning times up to a substantial fraction of a second or longer.
Recently, researchers have shown that alkaline earth sulfides have properties that can overcome at least some of these drawbacks. For example, in europium-doped magnesium sulfide (Eu2+:MgS), hole burning occurs when Eu2+ is photoionized to Eu3+ by two photons. A powerful laser is used for writing, providing the double photons, while a laser at much lower power is used for reading, with no destruction of the data. Indeed, 2000 read cycles failed to produce a measurable change in the hole-burned spectrum. At 2 K, 240 photon-gated holes have been burned and, in theory, this could be increased to 1000 holes. In addition, the holes are burned with single 10-ns pulses, leading to fast read/write rates. Finally, the holes seem stable at low temperature and have been observed to last at least 40 hours.
While such systems promise orders-of-magnitude improvement over existing data-storage technology, they remain far from commercialization and involve high-technology techniques such as liquid-helium cooling that would limit them to the highest-end applications. "We think that we can develop materials fairly soon that will allow us to reach liquid-nitrogen temperatures (77 K), which will make application much easier," explains Z. Hasan of Temple University (Philadelphia, PA), who has been active in researching Eu:MgS and related systems.
Moving toward practical systems
Other groups of researchers are looking at ways to move holographic systems much more swiftly into the market, albeit with more modest advances over conventional systems. A cooperative effort between researchers at Stuttgart and Berlin Universities has succeeded in developing a prototype disk storage system in which each spot on the disk is a microhologram.2 To use photopolymers that are stable at room temperature, the researchers limited frequency multiplexing to recording with six different colors, not using spectral hole burning. Combinations of red (633 nm), orange (612 nm), and green (543 nm) HeNe beams were used to produce the multiplexed holograms (see Fig. 2). Angle multiplexing is also used with 16 different angles for recording in a write-once/read-many system.While in these experiments, slow exposure times of about one second were used, photopolymers can be exposed with Nd:YAG laser pulses as short as 80 ps, so rapid recording seems feasible. In addition, the multiplexing scheme using 2-µm-diameter microholograms can produce data-storage densities of 100 bits/µm2, well beyond present digital-video-disk levels.
One step closer to commercialization is a packaged digital holographic data-storage system developed at Rockwell Science Center (Thousand Oaks, CA; see photo on p. 209).3 Here, Jian Ma and colleagues wanted rugged packages that could be used for avionics applications with no moving parts and fast data read out. To control spatial multiplexing, the system uses acoustic-optic deflectors. The storage medium, an iron-doped lithium niobate (Fe:LiNbO3) crystal, can store 1 Gbyte of data and has a 50-µs access time—considerably faster than a disk would have. The system achieves a total capacity of 10 Gbyte, with 1 Gbyte stored in each of 10 crystal slabs. The capacity of this system can be enhanced to about 50 Gbyte by increasing the page capacity to 1024 × 1024 and adding more crystal slabs. Each slab is 51 × 3 × 3 mm, so storage densities are around 7 bits/µm2.
Whether these near-term, room-temperature holographs, based mainly on spatial multiplexing, can successfully compete against rapidly evolving DRAMs and optical disks remains to be seen. If they can't, practical applications of holograms will have to await the perfection of spectral hole-burning techniques and a large jump in data-storage densities.
REFERENCES
- Z. Hasan, SPIE vol. 3468, 154 (July 1998).
- H. J. Eischler et al., IEEE J. Selected Topics in Quant. Electron. 4, 840 (Sept./Oct. 1998).
- J. Ma et al., SPIE vol. 3468, 8 (July 1998).
Eric J. Lerner | Contributing Editor, Laser Focus World
Eric J. Lerner is a contributing editor for Laser Focus World.