ADVANCED APPLICATIONS: BIOMEDICAL LASERS: Lasers support biomedical diagnostics
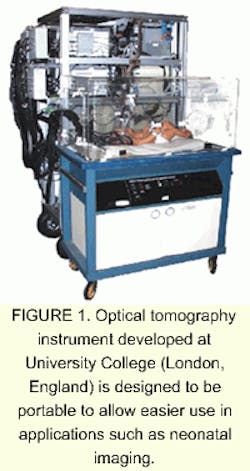
Lasers have long been used in medicine for surgery, with applications ranging from cauterization of blood vessels to drilling holes through the heart. Now, though, laser-based diagnostic devices are also proliferating in areas such as biomedical imaging and basic biological research. Ultrafast lasers are credited with making many of these applications possible.
Imaging for diagnostics
Perhaps the most spectacular advances are in the relatively new field of optical tomography, which uses ultrashort laser pulses to detect abnormalities within the body, instead of relying on potentially harmful ionizing radiation as other techniques do. The process works efficiently because human tissue is translucent to long-wavelength visible and near-infrared light, as is shown when a hand is held in front of a flashlight. Indeed, at a wavelength of 800 nm, tissue transmits one-third of the radiation over a distance of about 10 cm. Tissue appears opaque because it scatters light strongly, with a scattering length of only about a millimeter.
Because photons move through tissue in zigzag paths, in contrast to the straight-line routes taken by x-rays, optical tomography involves the difficult task of reconstructing an image from highly scattered photons. This is done with precision measurement of both the number of photons arriving in each direction and their time of arrival. With support from complex computer models that simulate how photons diffuse through an inhomogeneous medium, the data from an optical tomograph can be processed to detect changes in tissue optical properties that might, for example, indicate tumors.
One of the most ambitious optical tomography instruments to be developed is the Multichannel Opto-electronic Near-Infrared System for Time-Resolved Image Reconstruction, or MONSTIR.1 Developed at University College (London, England), the device has diverse applications ranging from the scanning of human breast tissue to measuring the heads of newborns (see Fig. 1). The instrument, which is contained in a 1.8 x 0.9-m-deep rack, sends picosecond-long pulses of light from a Ti:sapphire laser through a series of optical fibers, so that the point of illumination changes sequentially. The scattered light is collected by 32 bundles of optical fibers that feed into microchannel photomultiplier tubes. By recording the time of flight of each photon, the instrument builds up 1024 plots of photon path lengths, each representing the connection between a given point of illumination and a detector. After processing with specific image algorithms, these data yield images of the absorption and scattering patterns within the tissue. Currently, the device is still being tested in the laboratory.
A second type of laser imaging technique now entering clinical use is laser speckle flowimetry, which is used to detect changes in the blood flow within tissue. Here, laser light is scattered by moving blood cells, and its frequency is slightly Doppler-shifted. Other light is scattered from the static tissues with its frequency unchanged. When the two light waves interfere, the resulting speckle pattern varies relative to the blood flow. Rapid blood flow leads to a rapid variation in the speckle pattern, which can be measured by the detector.
Making fluorescence imaging easier
In biological research, an important trend in recent years has been the development of all-solid-state systems that bring high-performance lasers within reach of smaller research laboratories (see "Nanosensor makes bad cells quiver," below). One example of this is fluorescence lifetime imaging (FLIM). With this technique, short laser pulses in the femtosecond to picosecond range are used to excite fluorescence signals in biological tissues. The decay time of the return signal is measured, allowing the mapping of many biologically important substances such as oxygen and calcium.
Researchers at the Imperial College of Science Technology and Medicine (London, England) have developed a system for FLIM that is entirely solid-state, inexpensive, and portable. The operator does not have to be a laser specialist. The system generates 45-ps, 1-µJ pulses with a 5-kHz repetition rate at 860 nm.2 These pulses are then frequency-doubled to 430-nm pulses at 22 nJ. A CCD camera records the return fluorescence signal, and the computer measures the decay times for each pixel, fitting the decay curves as an exponential with fast and slow components. Different types of tissue exhibit different decay rates, so changes in tissue in response to medication can be easily mapped and measured (see Fig. 2).Laser surgery, while the oldest application of lasers in medicine, is also expanding in scope. One example is a new technique for relieving the pain of angina by laser-drilling holes in the walls of a heart.3 In the technique, termed transmyocardial laser revascularization, a surgeon uses a carbon dioxide laser to cut 15 to 30 1-mm-diameter holes through the wall of the heart. The outside surface seals immediately, but the channel remains open to provide oxygen-rich blood to previously deprived areas.
In a study of 192 patients done by researchers at Rush-Presbyterian-St. Luke's Medical Center (Chicago, IL), 72% of those treated improved significantly after a year (less angina pain), compared with only 13% of people treated only with medication. The improvement meant going from being bedridden with severe pain to moving about with little or no pain.
While angina surgery requires drilling relatively large holes, many other surgical applications require extreme precision. This is especially true for brain surgery, in which the damage to healthy cells must be minimized. Here, ultrafast pulses are useful because of their capability to avoid thermal damage. Unlike longer pulses, which heat tissue by conveying kinetic energy to atoms, ultrafast pulses deposit energy rapidly into the electrons, which disperse before the ions have time to heat up. The ions then fly apart without heating surrounding tissue.
In recent work at the Institute of Applied Physics, University of Heidelberg (Heidelberg, Germany), and the Department of Stereotactic and Functional Neurosurgery, University of Cologne (Cologne, Germany), researchers developed a computer-guided laser probe that delivers ultrafast laser pulses to precise spots in the brain.4 The probe consists of three coaxial tubes. The inner tube carries the laser beam to a focusing lens at its end. A second tube holds an elliptical deflecting mirror that can be rotated and moved axially, and the third tube, with a 5.5-mm diameter, fixes the position of the probe within the brain.
The device delivers 30-ps pulses of 1-µm radiation from a Nd:YLF laser to a 50-µm focused spot. Each pulse destroys less than one-thousandth of a cubic millimeter of tissue, allowing extremely precise control of the surgical process. With a 1-kHz pulse-repetition rate, about 50 mm3 of material can be removed per minute. The entire process is under PC control and relies on information from the surgeon related to the trajectory the probe will take and the region to be ablated. A laser scanning microscope operating through the same probe detects tiny blood vessels that are to be avoided. The system also can coagulate blood vessels that need to be cut.
Given the relative newness of affordable ultrafast lasers, these applications are likely to be only the beginning of a rapid expansion of new biotech laser uses.
REFERENCES
- J. C. Hebden et al., Opt. Lett. 24, 534 (June1999).
- R. Jones et al., Electron. Lett. 35(4), 256 (Feb. 18, 1999).
- O. H. Frazier, R. Marsh, and K. A. Horvath, New England Journal of Medicine 341,1021 (Sept. 30, 1999).
- M. H. Goetz, Phys.Med. Biol 44, N119 (May 1999).
Nanosensor makes bad cells quiver
A "nanoneedle" with a tip about one-thousandth the width of a human hair pokes a living cell, causing it to quiver briefly. Once withdrawn from the cell, the nanosensor detects signs of early DNA damage that can lead to cancer.
To simulate exposure to a carcinogen, the cell has been incubated with a metabolite of a chemical called benzo[a]pyrene (BaP), a known cancer-causing environmental agent often found in polluted urban atmospheres.1 Under normal exposure conditions, the cell takes up BaP and metabolizes it. The resulting metabolite reacts with the cell's DNA, forming a DNA adduct that can be hydrolized into a product called benzo(a)pyrene tetrol (BPT).
In the nanosensor, which was developed at Oak Ridge National Laboratory (ORNL; Oak Ridge, TN), the nanoneedle is a 50-nm-diameter silver-coated optical fiber that carries a helium-cadmium laser beam. Attached to the fiber tip are monoclonal antibodies that recognize and bind to BPT. The 325-nm laser light excites the antibody-BPT complex at the fiber tip, causing the complex to fluoresce. The newly generated light travels up the fiber into an optical detector. The layer of silver is deposited on the fiber wall to prevent the laser excitation light and the fluorescence emitted by the antibody-BPT complex from escaping through the fiber.
The nanonsensor was developed by a research group led by ORNL scientists Tuan Vo-Dinh, Guy Griffin, and Brian Cullum. The group believes that, by using antibodies targeted to a wide variety of cell chemicals, the nanosensor could monitor the presence of proteins and other species.
"Parallel arrays of these nanosensors could be used to detect gene expression and protein production in target cells," said Vo-Dinh. "They could also be used to screen tiny amounts of drugs to determine which ones are most effective in blocking the action of disease-causing proteins in single cells. With advances in nanotechnology, we are now approaching the ultimate limit of assessing the health of individual human cells."
REFERENCE
- ORNL Review 32, 14 (September 1999).
Eric J. Lerner | Contributing Editor, Laser Focus World
Eric J. Lerner is a contributing editor for Laser Focus World.