Waveguide Optics: Modular linear optical circuits can be chained to form complex information processors
Linear optical circuits consisting of transparent single-mode waveguides and other waveguide-based components can be used in interesting ways—for example, as all-optical neural networks. In fact, linear optical circuits have uses both in classical and quantum optical systems. One of the most interesting components in an optical circuit is the Mach-Zehnder interferometer (MZI), as it gives the circuit the ability to be reconfigured (in fact, this is the basis of some optical neural networks).
Up until now, all linear optical circuits have been made as monolithic devices, each designed with no particular thought to being modular. And, as with many other types of technical gear, modularity—with its repetitive manufacture of just one or a few types of components—promotes both flexibility and lower costs.
Scientists at the Optoelectronics Research Centre, University of Southampton (Southampton, England) the University of Oxford (Oxford, England), and Imperial College London (London, England) have created modular versions of linear optical circuits that can be butted up against each other to form larger optical circuits that are complex and highly reconfigurable because of the many MZIs in the circuit modules (see figure).1 The platform for the modular chips is ultraviolet-written silica on silicon.
Each module has a column of MZIs with phase shifters both on the input arms of and internal to the MZIs. In an experimental example, three modules have been concatenated to form a large, programmable interferometer with 20 inputs and outputs. Fiber-groove assemblies provide the inputs and outputs, and the microheater-based phase shifters (which alter phase based on a change in refractive index as a function of temperature) are controlled using electrical ribbon cables.
Modules make testing easier
The modules have advantages over large monolithic devices, say the researchers. First, testing and characterizing a small module with a single layer of MZIs is easier than characterizing a large, multilayered interferometer, and second, as many or as few layers of MZIs as desired can be concatenated together. However, the modular approach does have the disadvantage of higher coupling losses, which can be minimized with good mode-matching and the use of index-matching adhesive.
For couplers, the researchers chose an X-coupler design, in which incoming light evanescently couples between the modes for less than one-quarter of a sine wave while passing through the device. This design, say the researchers, has a low wavelength dependency, a small footprint, and reasonable fabrication tolerances.
The microheater phase shifters are stable and have a large tuning range, although they also have a slow tuning response of a few milliseconds. This response time is adequate for experimentation, though, the researchers note. Having a phase-shifter on each arm of each MZI improves stability and increases the tuning range by a factor of two.
Once fabricated, each module is characterized by measuring the emission from the two output ports of each MZI and the reflectance of the Bragg gratings embedded in the waveguides before, inside, and after the MZI. From this data, the splitting ratios, excess loss of the couplers, an estimate of the facet loss, and the phase shifters’ tuning curves can be determined.
Parameters measured at a 780 nm wavelength include a fiber-coupling loss of 0.8 ±0.1 dB, a chip-to-chip coupling loss of 0.2 ±0.1 dB, a propagation loss of 0.35 ±0.04 dB cm-1, a coupler excess loss of 2.1 ±0.3 dB, a phase-tuning range of (2.7 ±0.2) πrad, and a coupling ratio (transmittivity) of 57 ±4%.
Preliminary testing by the researchers showed that a three-module assembly could switch light from a single input to any of its six outputs (with some leakage) and could implement a balanced 3 × 3 interferometer (called a tritter) that can split light equally between all output ports. In addition, starting with randomly selected 3 × 3 unitary matrices, the actual transfer matrices were compared to the targeted transformations, showing an average transformation fidelity of 97.5%.
The researchers would next like to try out some quantum interferometric experiments on their modular devices.
REFERENCE
1. P. L. Mennea et al., Optica (2018); https://doi.org/10.1364/optica.5.001087.
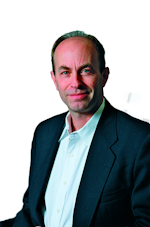
John Wallace | Senior Technical Editor (1998-2022)
John Wallace was with Laser Focus World for nearly 25 years, retiring in late June 2022. He obtained a bachelor's degree in mechanical engineering and physics at Rutgers University and a master's in optical engineering at the University of Rochester. Before becoming an editor, John worked as an engineer at RCA, Exxon, Eastman Kodak, and GCA Corporation.