Visible Laser Diodes: VI Systems develops room-temperature yellow laser diode emitting at 599 to 605 nm
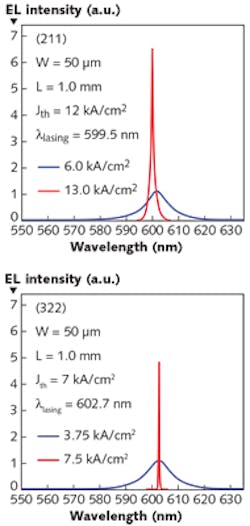
For laser diodes emitting in the visible spectrum, there has always existed a spectral gap in the yellow-orange region where room-temperature laser semiconductor materials can’t be made to emit via electrical pumping. This has now changed: researchers at VI Systems (Berlin, Germany), along with others from St. Petersburg Academic University and Ioffe Institute (both in St. Petersburg, Russia), Peter Grünberg Institut (Jülich, Germany), and CEMES–CNRS (Toulouse, France), have developed a laser diode that intrinsically emits in the yellow-orange spectral range from 599 to 605 nm wavelengths at room temperature in a laboratory environment.1
Laser diodes in the yellow-orange range can be used as compact wearable sources for medical blood control applications, eye surgery, in displays, industrial spectroscopic analysis, sensing, and compact optical atomic clocks with better than 10-16 frequency uncertainty.
The new device emits a narrow beam in the vertical direction (fast axis) with a full width at half maximum (FWHM) of 24°. A total of 0.8 W optical output power in pulsed mode operation was realized for a laser with a 1 mm cavity length and 50 µm stripe width, being limited by the catastrophic optical damage of unpassivated uncoated mirrors.
Commercially available laser-diode devices in this spectral range are based exclusively on frequency doubling of the laser light from an infrared laser diode by means of a nonlinear crystal.
Previous lab experiments with orange or yellow laser diodes based on various materials and geometries have resulted in either emission at or below 600 nm or room-temperature operation, but not both. The new laser diodes, which are based on aluminum gallium indium phosphide [(AlxGa1–x)0.5In0.5P] on GaAs substrates, achieve both these conditions simultaneously.
The new laser diode is fabricated so that it has four layers of tensile-strained InyGa1–yP quantum-dot-like insertions, which suppress leakage of nonequilibrium electrons from the gain medium. The layers between these insertions each were made with a different thickness to avoid any tunneling effects through resonant quantum-well energy levels.
The researchers grew experimental laser diodes on GaAs substrates with (211) and (322) crystal orientation. The structures grown on both substrates contain vertically coupled arrays of quantum dots that formed corrugated quantum-wire-like structures that had a lateral periodicity of about 35 nm.
Tilted-wave cavity
The researchers used a “tilted-wave” cavity configuration, which was developed by VI Systems and which allows for higher intracavity powers via a larger stripe thickness—the configuration reduces the chance of catastrophic end-facet damage. The stripe width of the devices was 50 µm and the cavity was 1 mm in length and had uncoated facets. The lasers were operated at 300 ns pulse duration and 1 kHz repetition rate.
Electroluminescence spectra below and above the laser threshold showed lasing for both types of devices, with an emission wavelength of 599.5 nm for the laser with the (211)-oriented substrate and 602.7 nm for the laser with the (322)-oriented substrate (see figure). The vertical and lateral far-field patterns were measured for the (211) laser, showing full-width at half-maximum (FWHM) emission angles of 24° and 2.4°. Because there was no passivation of the facets, the maximum optical output was limited to about 800 mW.
The researchers hope to eventually achieve green lasing at room temperature.
REFERENCE
1. N. N. Ledentsov et al., Opt. Express (2018); https://doi.org/10.1364/oe.26.013985.
About the Author
John Wallace
Senior Technical Editor (1998-2022)
John Wallace was with Laser Focus World for nearly 25 years, retiring in late June 2022. He obtained a bachelor's degree in mechanical engineering and physics at Rutgers University and a master's in optical engineering at the University of Rochester. Before becoming an editor, John worked as an engineer at RCA, Exxon, Eastman Kodak, and GCA Corporation.