ATTOSCIENCE: Photonic-crystal fiber produces attosecond pulses
A team of physicists has reported using a photonic-crystal fiber to manipulate light a million times more efficiently than previously known possible. The team consisted of physicists from the University at Bath (Bath, England), the Technical University of Denmark (Lyngby, Denmark), and the University of Oregon (Eugene, Oregon).
This discovery is also considered a milestone for the emerging field of attoscience—the ability to send out and measure pulses of light that last a mere billionth of one billionth of a second (see www.laserfocusworld.com/articles/294633 and 314415). These pulses are so incredibly fleeting that they enable researchers to more accurately measure the movement of subatomic particles. Attosecond technology may literally throw light onto the quantum world, where such particles have no definite position, only probable locations.
To generate attosecond pulses, the research team, led by Fetah Benabid at the University of Bath’s Department of Physics, created a broad spectrum of light, from visible wavelengths to x-rays, through an inert gas. This normally requires a gigawatt of power, but the team instead used a photonic-crystal fiber (PCF) to trap light and gas together. Until now, the spectrum produced by PCF has been too narrow for use in attosecond technology. But the team produced a broad spectrum using a Kagome lattice, using about a millionth of the power used by non-PCF methods.
So far, the effectiveness of PCF has primarily been its exploitation of the photonic bandgap, which stops photons of light from “existing” in the fiber cladding and traps them in the inside core of the fiber (see Fig. 1). The team used the fact that light can exist in different modes without strongly interacting, which results in a situation in which light can be trapped inside the fiber core without the help of photonic bandgap.
New theory of guidance
“We’ve developed a new theory of photonic guidance in hollow-core PCF,” Benabid explains. “The guidance is different from the now-conventional photonic-bandgap guidance, because it relates to the so-called ‘Von Neumann-Wigner’-bound and quasi-bound states within a continuum. That is, the hollow-core PCF exhibits the counterintuitive property of accommodating concurrently core-guided modes and cladding continuum modes-even with the same symmetry.”
At the heart of this discovery, the developed fiber exhibits transmission bandwidths several times wider than the state-of-the-art photonic-bandgap hollow-core PCF with relatively low loss. “The unique properties of this fiber made it possible to observe another breakthrough, which relates to the field of ultrafast optics where we demonstrated the generation and guidance of three-octave-spanning coherent spectral comb-like light (ranging in wavelengths from 325 to 2300 nm) using transient stimulated Raman scattering (SRS) in hydrogen-filled hollow-core PCF,” says Benabid (see Fig. 2). “In contrast with previous equivalent techniques, the spectrum is generated first, with power levels six orders of magnitude lower, and then with pulses five orders of magnitude longer in duration. The implications are wide-ranging. Our new photonic guidance theory may create a new direction for the design of next-generation photonic materials (that is, broadband hollow-core PCF and photonic crystals). In addition, the similarity between this guidance and the Von Neumann-Wigner states would constitute a new conceptual bridge between photonics and quantum mechanics.”And although the topic of multiline coherent SRS is a long-standing basic problem in nonlinear optical physics, Benabid believes his team’s new theory and experiments are significant steps forward in this area—stimulated by the availability of the new hollow-core fiber. “Indeed, the availability of ultrabroad coherent comb-like spectra that can be generated with very simple and moderately powerful lasers, and which covers several octaves at wavelengths spanning the UV to the IR, means that synthesizing attosecond pulses can be achieved more readily and at more ‘tamed’ wavelengths than those currently used (which lie in extreme-UV and soft-x-ray regions),” says Benabid.
The new techniques should also enable low-cost compact systems for subfemtosecond optical pulse generation—a development that should have a far-reaching impact on research in areas including laser science, materials science, and biological research.
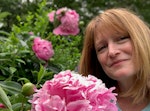
Sally Cole Johnson | Editor in Chief
Sally Cole Johnson, Laser Focus World’s editor in chief, is a science and technology journalist who specializes in physics and semiconductors.