ULTRAFAST OPTICS: Shaped pulses create fine illumination-field structure
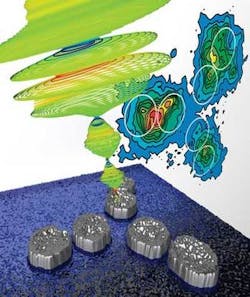
In research that lies at the border between nano-optics and coherent control, collaborators in Germany and Spain have demonstrated an adaptive pulse-shaping technique that spatially controls an illumination field at a scale much smaller than a wavelength. The result is a boost for near-field optics and may well open up a new length scale for optical imaging, quantum information processing, and spectroscopy.
The experiment was conceived by Tobias Brixner at the University of Würzburg (Würzburg Germany), Walter Pfeiffer at the University of Bielefeld (Bielefeld, Germany), and Javier García de Abajo from the Instituto de Optica (Madrid, Spain). The researchers start with 40 fs pulses from a standard Ti:sapphire oscillator. The pulses are then stretched and recompressed (but remain unamplified).
The key to the technique is a polarization pulse shaper at the Fourier plane of the compressor. Developed in 2001, the polarization shaper comprises two orthogonally oriented, 128-channel liquid-crystal-display (LCD) elements to control the relative phase of two transverse light polarizations.1 By manipulating the components of the dispersed light in the compressor, ultrafast modulations can be imposed on each successive pulse using the comparatively slow response of the LCD. Once incident on the sample, the two resulting local electric fields are no longer orthogonal and control of the relative phase between the two components controls their local interference. The phase angle between the two components is related to their relative location, giving the necessary tight spatial control.
The target, which was fabricated at the University of Kaiserslautern’s Nano+Bio Center, comprised an array of six silver disks lithographically fabricated on an indium tin oxide film. Each disk was 180 nm in diameter, with three pairs of disks forming a Y-shaped structure. By dusting the silver nanostructures with a 0.1-monolayer coating of cesium, the target’s work function was lowered just enough to permit two-photon emission under 790 nm excitation.
Learning algorithm
But how to measure the illumination on such small scales? The team invited Martin Aeschlimann at the University of Kaiserslautern and Michael Bauer from the University of Kiel to weigh in with their expertise on two-photon photoemission electron microscopy (PEEM). While monitoring the photoelectron emission from the sample, the researchers allowed a learning algorithm to adjust the polarization shaping of the incident pulses, thereby optimizing emission from various regions of the target-down to about a 180 nm resolution.2
Beyond being an impressive display of adaptive pulse shaping and another means to circumvent conventional resolution limits, the approach brings exciting applications into view. Time-resolving the PEEM signal would allow simultaneous control of spatial and temporal degrees of freedom, a result that Walter Pfeiffer says the group is now working on. “A flexible adaptation of the spatial and temporal properties of an emitted electron wave could in principle allow steering the propagating wave,” says Pfeiffer. “This might improve the temporal resolution of ultrafast electron sources that are limited to about 500 fs, or could find application in electron microscopy.” Better yet, such spatiotemporal control would be the first step toward a spectroscopy that several of the coauthors proposed in 2005.3 They envision the limits of standard pump-probe spectroscopy extended to a nanometer length scale and a femtosecond time scale, all from a single laser pulse.
While Pfeiffer admits that the technique will need some time to mature, there are a few nearer-term ideas. “The manipulation of supramolecular aggregates or of quantum-dot assemblies are particularly promising candidates,” he says. For the moment, the group is applying near-field optical theory to optical tweezers. “The first results indicate that control of the mechanical forces is feasible,” says Pfeiffer. “The tailored light fields might be indeed turned into actual mechanical tools.” The authors also mention the possibility of using the tailored fields to address nanoscale qubits in quantum information systems.
George Schatz of the Institute for Nanotechnology at Northwestern University (Evanston, IL) says that there has been growing interest in this kind of work, particularly from theorists who believe it is possible. Schatz is convinced that this demonstration will stimulate more work in this direction. “There are other methods for subwavelength imaging of electromagnetic interactions with metal nanostructures, but all have limitations,” Schatz says. “One could imagine that this technique could provide an imaging capability that could become popular.” However, he notes that there are a number of obstacles to overcome before the technique can graduate from specialist to being indispensable.
REFERENCES
1. T. Brixner and G. Gerber, Opt. Lett. 26 (8), 557 (2001).
2. M. Aeschlimann et. al., Nature 446, 301 (2007).
3. T. Brixner et. al., Phys Rev. Lett. 95, 093901 (2005).
About the Author
D. Jason Palmer
Freelance writer
D. Jason Palmer is a freelance writer based in Florence, Italy.