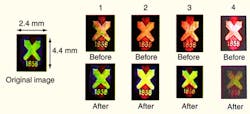
Researchers at Keio University (Yokohama, Japan), Koito Manufacturing (Sizuoka, Japan), and Cyber Laser (Tokyo, Japan) are developing a technique that allows holographic coherence gating to be extended to multicolor images. The new method, which uses a nonlinear photorefractive crystal as the holographic medium, is designed to keep image quality high despite the recording of multiple fringe patterns. The current setup is inadequate for many practical applications, particularly those related to biology and medicine. But the work could eventually lead to color systems that are faster, more flexible, and less noisy than had previously been considered possible.
Coherence gating is based on a simple principle: holograms can only be formed within the coherence length of the laser beam used. In a conventional holographic setup in which the available coherence length is short, the reference beam and object beam paths are carefully measured. If they are the same (within the tolerance of the coherence length), interference will take place. Outside that range, there will be no useful interference. As a result, the coherence length defines a depth window in the resulting hologram. When light is transmitted through a turbid medium as well as the object to be imaged, much of it is scattered (reflected many times). Each reflection increases the length of that beam path, lessening the likelihood that it will be sufficiently matched with the reference to interfere properly to form a hologram. Thus, only light that was relatively undeviated by the medium helps to form the image.
Multiple colors
Extending this technique to multiple colors is difficult because each wavelength travels through the same optical system and is recorded in the same volume of holographic material (in this case, a photorefractive crystal). This is a problem because the different holograms are competing for the same resource; each hologram partially erodes the others. For optical data storage, where the trick is to achieve the highest information density, a compromise is reached whereby each hologram is made so that, despite its erosion, it is clear enough to read the bits it contains sufficiently accurately. For imaging, however, this approach is inappropriate, because in this case image quality is more important than information density.For this reason, the Japanese researchers chose to record each hologram at a separate location (see Fig. 1).1 They achieve this by using a dispersive element—in this case a prism—to separate the different-color beam paths, both object and reference, so that volume holograms are formed at different locations within the crystal. The light can then be recombined on reconstruction so that a white-light view is produced.Though full-color imaging has been achieved, the technique is still relatively impractical for many applications (see Fig. 2). One of the biggest problems with the current system—which uses a continuous-wave helium cadmium laser to produce five red, green, and blue wavelengths—is that it is very slow. In one experiment, for instance, the holograms took 10 s to record. Another problem relates to the medium, which is only half as sensitive to green as it is to blue, and about a third again as sensitive to red. This means that fewer blue photons have more effect on the recorded interference pattern, thus reducing the blue signal-to-noise ratio. Because of these problems and others related to equipment, the demonstrator was limited to imaging through five mean-free paths in the turbid medium; however, further optimization of the optics, holographic medium, and sources used should both extend this depth and speed up the recording process.
REFERENCE
1. Masaki Ikuta et al., Appl. Opt. 41(10), 1882 (April 1, 2002).
About the Author
Sunny Bains
Contributing Editor
Sunny Bains is a contributing editor for Laser Focus World and a technical journalist based in London, England.