ULTRAFAST LASERS: Femtosecond amplifier output gets a boost
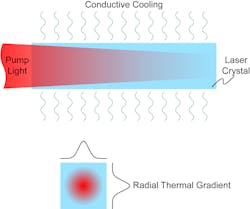
Numerous applications in applied physics and chemistry are driving a demand for ultrafast amplifiers that deliver femtosecond pulses at higher repetition rates and average power to increase signal-to-noise ratios and reduce data acquisition times. Delivering this performance without sacrificing pulsewidth, beam quality, operational simplicity, or reliability has proven to be a challenge. Recent design improvements now enable a system that yields up to 15 W of average power using only thermoelectric (TE) cooling of the Ti:sapphire gain medium. Previously, these output power levels required cryogenic cooling, resulting in complex and space-consuming systems.
The need for power
The Ti:sapphire-based modelocked laser oscillator revolutionized applications of ultrafast lasers, simplifying access to femtosecond pulses. A typical Ti:sapphire oscillator delivers average powers up to 2–4 W. At repetition rates of 50–100 MHz, the pulse energy is at most a few tens of nanojoules, with a peak power of about 500 kW, depending on the pulse duration.
Many applications require much higher pulse energy or peak power, a need met with chirped pulse amplification (CPA). The oscillator pulses are stretched, selected by an appropriate pulse picking device, amplified by several orders of magnitude in another Ti:sapphire crystal, then recompressed to pulsewidths almost as short as the initial pulses.
Amplified femtosecond pulses can be used to pump one or more tunable optical parametric amplifiers (OPAs) for pump-probe studies in solid-state physics and photochemistry. They can also be used to generate THz pulses for imaging or spectroscopy. More recently, the availability of amplified systems featuring carrier to envelop phase (CEP) stabilization opened the door to the production of attosecond pulses at extreme UV (4–30 nm) wavelengths—pulses that are short enough to study the dynamics of electrons in atoms and molecules.
Many of these applications involve one or more cascaded or parallel nonlinear conversion stages (parametric conversion or other effects) that are often relatively inefficient. Thus, increasing the amplifier energy per pulse offers the capability to efficiently drive multiple, high-energy, nonlinear stages in parallel.
Thermal lensing
A major technical hurdle in scaling ultrafast amplifier systems to higher power is thermal lensing (distortion) in the gain crystal. Even with laser pumping, more than 75% of the pump power is converted into heat. End-pumping the Ti:sapphire rod creates a radial power distribution where maximum pump intensity is delivered along the center line of the gain medium. Local heating in the Ti:sapphire crystal follows the intensity pattern of the pump light and the crystal is conductively cooled via its mounting surface(s). The end result is a radial thermal gradient perpendicular to the direction of the laser beam (see Fig. 1). Even a pump power of just 10 W and a beam waist of 50 μm, for instance, can result in a thermal lens of more than 50 diopters. With pulsed pump lasers available at 40 W and higher, this lensing effect becomes the single largest design challenge.
There are several ways to cool a Ti:sapphire crystal, thereby reducing the strength of the thermal lens effect. Unfortunately, the effectiveness of these cooling methods scales with their cost and complexity. Passive conductive cooling is the simplest method, followed by water cooling. These approaches are practical for powers up to 3–4 W. In higher-performance systems, the Ti:sapphire crystal is usually cooled with a TE cooler, but until recently even with this method the maximum average power was 7–8 W.
The ultimate approach is to use cryogenic cooling. At these low temperatures the thermal conductivity of Ti:sapphire increases roughly 40 times and the dependence of refractive index on temperature drops by an order of magnitude. The net result of these two effects is about a 400 times decrease in thermal lens power between a Ti:sapphire crystal at 300 K and one cooled to 77 K. Until now, all amplifiers producing more than a few watts were cooled cryogenically, with disadvantages in terms of cost and complexity.
A 15 W system
Diverse applications concur to make highly desirable a 15 W class amplifier with the same simplicity and beam quality as a 3–4 W system.
We chose a hybrid design to deliver the beam quality of a regenerative amplifier without the cost and complexity of cryogenic cooling. Specifically, the Coherent Legend Duo HP uses a regenerative amplifier followed by a single-pass amplification stage, all in the same box, with TE cooling for both Ti:sapphire crystals.
This approach is different from multipass amplification where the input pulses pass about 10–15 times through the crystal, with each pass at a slightly different angle but providing some overlap with the pump beam. Such a system is optically complex and results in beam quality and pointing that is degraded compared to the seed laser beam quality. In contrast, a regenerative amplifier is a high-gain laser cavity where pulses from the seed laser are amplified during numerous round-trips and, upon reaching gain saturation, are coupled out of the cavity (see Fig. 2). The output beam characteristics of the amplifier are independent of the seed laser, governed by the simple cavity design, and produce a near-perfect TEM00 mode with stable pointing characteristics suited for downstream nonlinear generation processes. The additional single-pass power amplifier is simple and maintains the beam quality of the regenerative amplifier.Ultrafast amplifiers are usually selected based on parameters like energy per pulse, repetition rate, and mode quality. As the experiments become more complicated, turnkey reliability and ease of use become increasingly important. Design refinements make it possible to improve flexibility and performance, along with simplicity and turnkey operation.
Steve Butcher | Scientific Marketing Manager, Coherent
Steve Butcher is scientific marketing manager at Coherent (Santa Clara, CA).
Marco Arrigoni
Marco Arrigoni is vice president of marketing at Light Conversion (Vilnius, Lithuania). He previously served as director of marketing at Coherent (Santa Clara, CA) from 2007 through 2023.