PHOTONICS APPLIED: FREE-SPACE COMMUNICATIONS: Sophisticated optical systems extend reach of free-space communications
Since the earliest demonstrations of free-space optical (FSO) communications for defense applications in the 1960s, steady improvements in light sources, detectors, and optical components continue to extend the reach for FSO networks, as well as increase the wish list for possible applications. For example, the same University of Padua (Padua, Italy) researchers who demonstrated free-space transmission of single photons over a distance of 1500 km in 2008 have, just two years later, proposed a space-based quantum-key-distribution (QKD) terminal for the International Space Station.1
Space-borne communications
Although the Mars Laser Communication Demonstration project was scrapped in 2005, space-borne FSO communications are alive and well.
In 2006, ARTEMIS, the European Space Agency (ESA) Advanced Relay and Technology Mission Satellite in its geostationary position at 36,000 km altitude, relayed an optical signal from its SILEX laser link to an aircraft flying at altitudes of 6 and 10 km-a feat equivalent to targeting a golf ball over the distance between Paris and Brussels.2 "The bandwidth potential for FSO communications is two orders of magnitude greater than RF or microwave communication," says Stefano Badessi, applications engineer at ESRIN (Frascati, Italy), the ESA Centre for Earth Observation (see Fig. 1).
Institutions such as the Massachusetts Institute of Technology's (MIT) Lincoln Laboratory (Lexington, MA) used a superconducting nanowire single-photon detector (SNSPD) to realize an error-free photon-counting communication link at a data rate of 781 Mbit/s (the fastest at that time in 2006). "Today, we are in the process of building a free-space communications system for NASA between a satellite in lunar orbit and a ground-based telescope array," says Andrew J. Kerman, technical staff member at MIT Lincoln Laboratory. "This Lunar Laser Communications Demonstration (LLCD) program will serve as a pathfinder to illustrate the potential of photon-counting laser communications to NASA. Its ground receiver will use four quad nanowire arrays and support data rates up to 0.6 Gbit/s with only a few hundred milliwatts of power at the lunar transmitter."3
In addition to improved detection schemes, source wavelength and modulation format are also critical to successful FSO communications.4 Scientists at Discovery Semiconductors (Ewing, NJ) in partnership with several other research groups built a satellite FSO communications system that transmits 10.7 Gbit/s at 1.55 μm over a distance of several tens of thousands of kilometers using return-to-zero differential phase-shift keying (DPSK) modulation.
"The German satellite TerraSAR-X carries a coherent optical high-speed communications terminal as secondary payload, built by Tesat-Spacecom [Backnang, Germany], under DLR contract," says Hennes Henniger at the German Aerospace Center (DLR; Wessling, Germany). The terminal transmits at 5.6 Gbps up to 6000 km in space with 700 mW transmit power using coherent binary phase-shift keying (BPSK) homodyne reception, and has been used to successfully demonstrate inter-satellite links with the LEO satellite NFIRE and downlinks to ground stations.5
Closer to home, DLR spinoff ViaLight Communications (Gilching, Germany) specializes in FSO networks from the ground to unmanned aerial vehicles (UAVs), aircraft, or high-altitude platforms. These compact and "mobile" FSO networks have typical 1 Gbit/s speeds with 100 km reach (see Fig. 2). But unlike the vacuum of space, terrestrial FSO networks are challenged by ever-changing atmospheric conditions.Terrestrial links
"Originally developed for secure military communications, FSO networks have improved tremendously and continue to leverage their 'covert' strengths," says Heinz Willebrand, CEO of LightPointe (San Diego, CA) (see Fig. 3). "Typical FSO beam divergence is on the order of 2-5 mrad; translation: the beam spot is only 2-5 m at a 1 km distance compared to a radio antenna that can spread out the signal over 100 m or more at the same distance. Also, there is an enormous amount of 'free' spectrum available in the optical communications band. While unlicensed and licensed RF and microwave systems up to roughly 40 GHz typically operate over spectrum bands that are very limited in size-20, 30, 50 MHz, for example-the spectrum available in the optical communications band exceeds several 100 GHz!"LightPointe's FSO systems operate at speeds up to 1.25 Gbit/s in full-duplex mode using multiple transmitters and receivers. "This increases not only the power we can launch from the terminal but also increases the overall receive lens surface while minimizing the impact of 'heat shimmer' or 'scintillation' that can negatively impact the bit-error-rate [BER] performance of longer-distance FSO systems," adds Willebrand.
Indeed, while many terrestrial FSO systems use single-laser sources between 780 and 850 nm, multisource systems can improve transmission when atmospheric turbulence causes scintillation and fading or when clouds and aerosols cause scattering and pulse broadening. "For FSO systems operating outdoors, we have developed mitigation techniques incorporating multiple transmit lasers and multiple receive apertures," says Mohsen Kavehrad, W.L. Weiss professor of electrical engineering at Pennsylvania State University (University Park, PA).6 "The main concept of these MIMO [multi-input multi-output] systems is to use the spatial diversity available from scintillation prevalent in long links, to improve received signal-to-noise ratio and BER. As a result, it is possible to sustain longer links with the same amount of power used by a single-input single-output [SISO] system." Kavehrad adds, "Adaptive optics is another solution to compensate for phase perturbation on long-distance links."
Boston Micromachines (BMC; Cambridge, MA) offers both microelectromechanical systems (MEMS) deformable mirrors for aberration correction and MEMS modulating retroreflectors (MRRs) for FSO covert communication and remote sensor integration. Originally developed for a DARPA coherent laser communication project, their Kilo-DM deformable mirror has 1020 actuators controlled to <1 nm of precision with no hysteresis. "The Kilo-DM can pre-correct for distortions present in the beam path as well as correct for defects in the optical systems used to transmit or receive data," says Michael Feinberg, director of product marketing at BMC. And applicable to both terrestrial and space-borne applications, BMC's MRR uses a MEMS modulator mounted in a corner-cube retroreflector for asymmetric FSO communications at 180 kHz transmission speeds (see Fig. 4). "The term 'asymmetric' essentially means 'one way': in this case, our MRR receives an interrogating beam and modulates the reflected beam to transmit information," says Feinberg. "Bidirectional communication is also possible if beams are projected from two nodes with corresponding MRRs at each node."MRV Communications (Chatsworth, CA), who has installed more than 8000 terrestrial FSO links, uses multiple 100 mW infrared transmitters in its TereScope system and an 8 in. diameter receiver for data rates up to 10 Gbit/s at distances up to 5.5 km in a variety of weather conditions. In light rain and haze, transmission signals typically see 3 dB/km attenuation values that increase to 17 dB/km for cloudbursts and medium snow, and are as high as 30 dB/km in the presence of steady rain, a blizzard, or light fog.
Longer wavelengths, longer reach
"Because Rayleigh scattering diminishes with increasing wavelength, a 10 μm wavelength source offers significantly less scattering than a 1.55 μm wavelength," says Kumar C. Patel, CEO at Pranalytica (Santa Monica, CA). Patel references a Stevens Institute of Technology (Hoboken, NJ) study that showed 2X to 3X greater transmission during fog formation and after a short rain event (that reduced visibility to approximately 1 km) using an FSO system with an 8.1 μm source compared to using conventional 1.3 and 1.5 μm sources.7 "We are working on FSO communications using mid-infrared [mid-IR] radiation sources-specifically quantum-cascade [QC] lasers—and developing new detection systems that allow for easy demodulation of the signal," says Rainer Martini, director of the Laboratory for Ultrafast Spectroscopy and Communications at Stevens Institute of Technology.
Daylight Solutions (San Diego, CA) is also exploring the functionality of FSO communications using mid-IR QC lasers through a collaboration with San Diego State University. While these 3-12 μm sources are excellent at identifying the spectral "fingerprint" of atmospheric molecules, FSO transmission is only successful if the launch wavelength avoids the atmospheric absorption lines of water vapor, CO2, and pollution or other common atmospheric molecules. "For this reason, longer wavelengths between 8 and 12 μm are considered optimal candidates for next-generation terrestrial FSO communications networks," says Sam Crivello, senior scientist at Daylight Solutions. "Our tunable external-cavity QC lasers allow the user to tune to a window in a dynamically changing atmosphere to avoid the interference of pollutants and water, for full-duplex communications with high baud rates and very low BERs using wavelength-division multiplexing techniques."
Undersea challenges, future terrestrial promises
In early 2010, the Woods Hole Oceanographic Institution (WHOI; Woods Hole, MA) developed an optical/acoustic undersea communications system that transports data over a visible-light beam at 1-10 Mbit/s data rates at 100 m distances from untethered remotely operated vehicles (ROVs) to surface ships or laboratories. Acoustic communications then take over at distances beyond 100 m.
From satellite-to-satellite links in space to undersea ROV exploration, FSO communications will thrive as transmit/receive technologies improve and new applications emerge. "FSO is still a niche market with most applications in the enterprise/building-to-building connectivity space," says Willebrand of LightPointe. "However, next-generation mobile wireless networks will have to provide a lot of access capacity to a single handset/smart phone and to do this, distances between microcell sites are coming down to below 500 m-exactly the 'sweet spot' for high-capacity FSO systems," he adds. "The deployment of FSO technology in mobile wireless backhaul networks should boost the overall total addressable market for FSO quite dramatically."
Already, companies like fSONA (Richmond, BC, Canada) are deploying their 1550 nm eye-safe SONAbeam FSO networks to support wireless backhaul and broadband applications. "Every year, the fiber-optics industry invests billions of dollars developing new components and subsystems for the 1550 nm region," says fSONA CTO Paul Erickson. "1550 nm diode lasers that operate at 2.5 Gbit/s are already available, with 10 Gbit/s devices beginning to appear. In addition to higher possible speeds, the output power for 1550 nm FSO systems is up to 50X higher than for 800 nm systems, allowing operation over longer distances and/or through heavier fog attenuation with greater reliability."
REFERENCES
1. P. Villoresi et al., New Journal of Physics, 10, 033038 (2008).
2. http://telecom.esa.int/telecom/www/object/index.cfm?fobjectid=27945
3. E.A. Dauler et al., J. Modern Opt., 56, 2, 364-373 (2009).
4. I.B. Djordjevic, J. Optical Comm. and Networking, 2, 5, 221-229 (2010).
5. S. Das et al., MILCOM 2008 Unclassified Program Session: Next Generation Networks and Systems, paper 1317, San Diego, CA (November 2008).
6. Z. Hajjarian et al., J. Comm., 4, 8, 524-532 (September 2009).
7. P. Corrigan et al., Opt. Exp., 17, 6, 4355-4359 (March 16, 2009).
About the Author
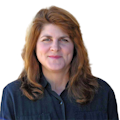
Gail Overton
Senior Editor (2004-2020)
Gail has more than 30 years of engineering, marketing, product management, and editorial experience in the photonics and optical communications industry. Before joining the staff at Laser Focus World in 2004, she held many product management and product marketing roles in the fiber-optics industry, most notably at Hughes (El Segundo, CA), GTE Labs (Waltham, MA), Corning (Corning, NY), Photon Kinetics (Beaverton, OR), and Newport Corporation (Irvine, CA). During her marketing career, Gail published articles in WDM Solutions and Sensors magazine and traveled internationally to conduct product and sales training. Gail received her BS degree in physics, with an emphasis in optics, from San Diego State University in San Diego, CA in May 1986.