LASER PHYSICS: Lasers accelerate electrons in three approaches
Research teams in England, the United States, and France have independently achieved breakthroughs in plasma-based particle accelerators that could eventually lead toward realization of a tabletop particle accelerator. Conventional particle accelerators must be hundreds of meters in length to accelerate electrons to giga-electron-volt energies, whereas laser-produced plasmas can support electric fields thousands of times greater than those of conventional accelerators in a very small space. Unfortunately, it has only been possible to accelerate particles in plasma-based accelerators over a distance of approximately 1 mm with resulting beams having a large energy spread. By exploiting variations of laser wakefield acceleration, the teams have produced monoenergetic electron beams with an energy spread of less than a few percent.
Using the Astra laser at the Rutherford Appleton Laboratory (Didcot, England), researchers were able to focus 40-fs, 0.5-J, 800-nm laser pulses onto the edge of a supersonic jet of helium gas to produce peak intensities up to 2.5 × 1018 W/cm2. The ponderomotive force of the laser—the force that causes electrons in the plasma to be pushed ahead of the laser pulse—causes a plasma wave that continually increases in amplitude. When the wave breaks, the background electrons are trapped and boosted to high energy.
Because this laser wakefield acceleration is several plasma wavelengths in duration, successive plasma periods can accelerate trapped electron bunches to different energies. By carefully controlling the plasma density or through forced laser wakefield acceleration, only the first plasma oscillation is driven to the breaking point if the laser pulse length is less than the plasma wavelength. All of the electrons in this first bunch experience very similar acceleration to values of 50 to 80 MeV and an energy spread of less than 3% (see figure).1
In contrast to the concept of forced laser wakefield acceleration, researchers at Lawrence Berkeley National Laboratory (Berkeley, CA) instead created a plasma channel to guide the ultra-intense laser pulses and shape the plasma wakes to create monoenergetic electron beams. The plasma channel was formed in a hydrogen-gas jet by two laser pulses fired 500 ps before the driving pulse was applied from a Ti:sapphire laser at 810 nm. The plasma was then heated to tens of electron volts, expanding outward and creating a shock wave that resulted in plasma density depletion over a 2-mm length. The resultant beam had a spread of ±2% with a peak of 86 MeV.2
Finally, researchers from the Ecole Polytechnique (Palaiseau, France) have focused a compact, chirped-pulse-amplification laser onto a helium gas jet and, again using the ponderomotive force of the laser, have observed that the plasma electrons are expelled and leave a cavitated region or “bubble” behind the laser pulse. As the electron density at the walls of the bubble becomes large, wave-breaking occurs and more and more electrons are accelerated inside the bubble, causing the bubble to elongate and bunching up the electrons, producing a monoenergetic peak in the energy spectrum of up to 170 MeV.3
All three research teams attribute the advances in plasma-based particle accelerators to improvements in the lasers used to deliver the intense pulses required for laser wakefield acceleration.
REFERENCES
- Stuart Mangles et al., Nature 431, 535 (Sept.30, 2004).
- C. G. R. Geddes et al., Nature 431, 538 (Sept. 30, 2004).
- J. Faure et al., Nature 431, 541 (Sept. 30, 2004).
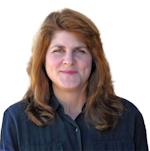
Gail Overton | Senior Editor (2004-2020)
Gail has more than 30 years of engineering, marketing, product management, and editorial experience in the photonics and optical communications industry. Before joining the staff at Laser Focus World in 2004, she held many product management and product marketing roles in the fiber-optics industry, most notably at Hughes (El Segundo, CA), GTE Labs (Waltham, MA), Corning (Corning, NY), Photon Kinetics (Beaverton, OR), and Newport Corporation (Irvine, CA). During her marketing career, Gail published articles in WDM Solutions and Sensors magazine and traveled internationally to conduct product and sales training. Gail received her BS degree in physics, with an emphasis in optics, from San Diego State University in San Diego, CA in May 1986.