QUANTUM PHYSICS: Semiconductor chip converts laser to visible quantum fluid
University of Cambridge (Cambridge, England) researchers can see quantum mechanics at work with the naked eye. They have developed a semiconductor chip that tightly traps laser light in the vicinity of electrons inside microscopic cavities, producing polaritons—quasi-particles created by mixing semiconductor excitons with microcavity photons—that enable direct visualization of quantum states of light.1
Superfluidity
The 1 × 10 cm chip consists of a 5λ/2 period aluminum gallium arsenide (AlGaAs) distributed Bragg reflector (DBR) microcavity with four sets of three quantum wells placed at the antinodes of the DBR cavity electric field. The cavity quality factor Q exceeds 8000, and illumination from the two pump lasers is chopped at 100 Hz to avoid heating and input directly to the chip through a 0.7 numerical-aperture lens. The chip is held at cryogenic temperatures below 50 K, and images are recorded on an uncooled silicon CCD in a magnified image plane.
When the semiconductor chip is illuminated with two or more 1-μm-diameter spots of pump-laser light at 750 nm, two-dimensional polariton condensates form a spontaneously oscillating quantum fluid that allows visualization of quantum mechanics in action. The effects are possible because the polaritons, which Bose-condense above a certain threshold, feel an outward force and form an expanding polariton condensate that interacts with the opposing condensate from the other pump spot.
Naked-eye visibility
As the two polariton (quasi-particle) condensates interact, the interference effects differ depending on the pump intensity and the spacing between the two (or more) pump sources on the chip. On the line between pump spots, the polaritons experience a potential that causes them to redistribute as if trapped in a simple harmonic oscillator, occupying different oscillator states as the spacing between pump spots is varied (see figure).
The sloshing of coherent polaritons back and forth at tunable terahertz frequencies is visualized using time-delayed interferometric combination of images of the superfluid quantum states.
“Controlling the motion and better understanding the behavior—and quantum-mechanical entanglement—of this quantum fluid could lead to a new generation of ultrasensitive gyroscopes to measure gravity, magnetic fields, and create quantum circuits,” says postdoc member Gabriel Christmann at the University of Cambridge. “It is amazing to see quantum mechanics in front of your eyes on this macroscopic scale as big as a human hair.”
REFERENCE
1. G. Tosi et al., Nature Phys. online (Jan. 10, 2012); doi:10.1038/nphys2182.
About the Author
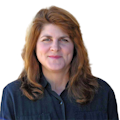
Gail Overton
Senior Editor (2004-2020)
Gail has more than 30 years of engineering, marketing, product management, and editorial experience in the photonics and optical communications industry. Before joining the staff at Laser Focus World in 2004, she held many product management and product marketing roles in the fiber-optics industry, most notably at Hughes (El Segundo, CA), GTE Labs (Waltham, MA), Corning (Corning, NY), Photon Kinetics (Beaverton, OR), and Newport Corporation (Irvine, CA). During her marketing career, Gail published articles in WDM Solutions and Sensors magazine and traveled internationally to conduct product and sales training. Gail received her BS degree in physics, with an emphasis in optics, from San Diego State University in San Diego, CA in May 1986.