Optogenetics/Cell Biology: Beyond the brain: Non-neural optogenetics
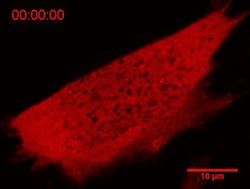
It is customary to think of optogenetics, the application of light to control cellular activity by way of encoded genes, as a neuroscience technique. In fact, optogenetics was developed for neuroscience research, and the best-known optogenetics pioneers, Karl Deisseroth at Stanford and Ed Boyden at MIT, work in this area. Researchers at their labs and others have amply demonstrated the technique in experiments on mice, effecting behavior by activating genes that express light-sensitive ion channels. When cells of interest express genetically encoded molecular reagents, scientists can control specific biological processes with great precision.1
But such control is not limited to neurons, and it turns out that neuroscientists aren't the only ones to recognize the tremendous potential of being able to interact with intracellular structures and signaling pathways. A newer, and quickly growing, direction in optogenetics involves working with non-neuronal cells. For this type of work, traditional cell manipulation methods (chemical approaches and genetic "knockout") require long periods of time for observation and are not reversible. By contrast, optogenetics provides a high degree of spatiotemporal precision and reversiblility, meaning, for instance, that scientists are able to switch proteins on and off to compare effects.
Thus, optogenetics promises new insight into molecular mechanisms important on multiple levels—mechanisms that influence the development of form and structure, as well as ongoing operation, in both cells and whole organisms. The technique enables the dissection of complex signaling networks, and carries less risk of unintended side effects.2 What's more, optogenetics is relatively inexpensive to use.
Different cells, different tools
While neuronal optogenetics involves control of "channel proteins," work in cellular and developmental biology involves other processes that affect cell signaling—and thus requires a different toolbox.3 So it is not surprising that much of the work in non-neuronal optogenetics has been centered on the development of tools and systems to enable the exact types of manipulation needed to answer researchers' burning questions.
But when scientists who use optogenetics say the words "tools" and "systems," they generally mean light-activatable proteins. Scores of journal papers have reported the design of a specific agent and its application to answer a particular question; others have summarized the development of these agents. And recently, a startup called Optologix, developer of light-activated tools promising cell biologists the ability "to probe cellular behavior with unmatched spatial and temporal control," introduced its LITE Switch Kit, "the first commercially available light-inducible gene expression system."
In fact, while optogenetics relies on optics and photonics, attention to these tools has been limited. That's because optogenetic agents tend to be sensitive to visible light, so common microscopy light sources—including light-emitting diodes (LEDS) and lasers operating at low power—can activate them. Low-magnification objectives enable stimulation of single cells, high-magnification objectives can be used to activate subcellular regions, and digital micromirror devices (arrays of mirrors that can be individually controlled to project specific light patterns) enable fine spatial control and dynamic response changes in cell shape.4
However, "digital micromirror devices for patterned light stimulation are expensive and seen as a niche product, and we don't have cheap, robust light delivery devices for mounting in incubators or on various multi-well plates," says Jared Toettcher, assistant professor of molecular biology at Princeton University. "Most of these light delivery devices are still built à la carte in the labs that are doing the work."
And unlike neuroscience experiments, which require miniature headsets to deliver light into the brains of behaving mice, optogenetics-based cell biology requires no bespoke hardware.
Open-source agent comparison
Researchers at Rice University (Houston, TX) have another idea for expanding adoption: They say that the impact of optogenetics and photobiology has been limited by a lack of optical hardware to enable proper use of non-neural agents. "There is little data directly comparing the performance features of optogenetic tools. Accordingly, researchers may have difficulty selecting the best tool for a given experiment," the Rice team reports.
So, the Rice research team has designed an open-source, build-it-yourself hardware-software solution. Their creation promises to enable direct, quantitative comparison—on the basis of multiple properties (spectral, intensity-dependent, and dynamical)—of all agents providing gene expression outputs-in diverse organisms (bacteria, yeast, mammalian cells, and more). Using the setup in their own experiments, they found, for instance, that one agent is 50 times less sensitive to light than another. The availability of this type of information, they say, should accelerate adoption of optogenetics.
The hardware part of the system, called Light Plate Apparatus (LPA), is a LED-based device that costs less than $400 in parts (see Fig. 1). Its consumable costs are similarly low. LPA accommodates a 24-well plate, and delivers a pair of independent light signals to each of the wells with millisecond resolution. Users can program the signals using a web-based interface called Iris, which allows control over wavelength (310–1550 nm) and intensity (over three orders of magnitude). The scalable system offers high throughput, and the team says that a non-expert user can assemble and calibrate LPA in a single day. Because both the hardware and software are open-source, scientists can freely modify and extend them for additional uses.Microscopy enablers
Still, this nascent application of optogenetics is already producing results. "The field is moving beyond proof of concept to answering real biological questions, such as how cell signaling is regulated in space and time, that were difficult or impossible to address with previous tools," explains Doug Tischer and Orion Weiner of the University of California San Francisco's Cardiovascular Research Institute and Department of Biochemistry and Biophysics.
And, according to Weiner, more elegant integration of optics and photonics-based instruments—and, ideally, automation—would help advance non-neural optogenetics research. "There aren't great seamless integrations of hardware/software that enable users to specify a given series of light inputs (that are varied in time and concentration) interleaved with the fluorescence-based imaging platforms," he says.
Even better would be microscopy systems that "could be programmed to make decisions during the experiment," Weiner adds. He'd like an instrument to scan a sample in search of cells expressing components of interest and activity readouts (the way your car radio scans for viable signals), and then, when it finds an appropriate target, attempt preliminary optostimulation to determine whether the cell is responsive. Assuming it is, the system could then perform a pre-programmed experiment. If it's not, it would continue to scan for another likely candidate, repeating the process until either it has collected a predetermined number of cells, or all cells in the sample have been judged to be overgrown or nonresponsive.
During a five-day practical course called Non-Neuronal Optogenetics: From Design to Application in Cell Signaling and Tissue Morphogenesis, given at the European Molecular Biology Lab in June 2016, Dr. Valentina Emiliani of the European Neuroscience Institute (Paris, France) presented the work of her wavefront-engineering microscopy team, which is developing optical techniques based on spatiotemporal engineering of optical wavefronts using phase modulation. The approach promises to minimize power losses, quickly adapt excitation patterns to an experiment, and enable 3D sculpting of excitation volumes.
Toward clinical application
Commenting on recently reported research done at Princeton, Toettcher's colleague, assistant professor of chemical and biological engineering Clifford Brangwynne, says, "This is fundamental science we're doing, answering basic questions about phase transitions in cells." The ultimate goal, however, is larger: "we're hoping these insights will reveal not only how healthy cells work, but also how they can become diseased, and maybe eventually cured."5 Indeed, many applications that promise clinical value beyond the brain are emerging (see image at top of this page).
So far, the optogenetics-based work that has made the greatest progress toward clinical application is RST-001, an agent designed by Wayne State University (Detroit, MI) researcher Zhuo-Hua Pan (who may in fact be the originator of the optogenetics technique, according to a September 2016 article in STAT, a Boston Globe Media publication) for treatment of retinitis pigmentosa (RP). After securing a patent to use channelrhodopsin-2 for vision restoration, Pan licensed it to a company called RetroSense Therapeutics (Ann Arbor, MI), which was acquired by global pharmaceutical company Allergan in late 2016 in an all-cash transaction starting with a $60 million upfront payment, and involving potential regulatory and commercialization milestone payments.
RST-001 creates new photosensors in retinal ganglion cells: The FDA cleared RetroSense's Investigational New Drug (IND) application in August 2015, and the company reported success with a Phase I/IIa clinical trial to evaluate RST-001's safety a year later. Allergan chief research and development officer David Nicholson said in a statement, "The RST-001 program and its optogenetic gene therapy approach could be a real breakthrough in the treatment of unmet needs across a host of retinal conditions, including RP," adding that "Allergan is excited by the prospect of advancing an entirely new approach."
Another example of the potential for clinical application of non-neuronal optogenetics is detailed in a paper titled, "A Synthetic Erectile Optogenetic Stimulator Enabling Blue-Light-Inducible Penile Erection."6
Even if non-neural efforts account for only a minority of work being done in the roughly 1000 laboratories worldwide doing optogenetic research (according to Credence Research's study, "Optogenetics Market Growth, Future Prospects and Competitive Analysis, 2016-2022"), the field has an interesting future.
REFERENCES
1. B. Y. Chow and E. S. Boyden, Sci. Transl. Med., 5, 177ps5 (2013).
2. K. Zhang and B. Cui, Trends Biotechnol., 33, 2, 92–100 (2015).
3. D. Tischer and O. Weiner, Nat. Rev. Mol. Cell Biol., 15, 8, 551–558 (2014).
4. K. P. Gerhardt et al., Sci. Rep., 6, 35363 (2016); doi:10.1038/srep35363.
5. Y. Shin et al., Cell, 168, 1–2, 159–171.e14 (2017).
6. T. Kim, M. Folcher, M. Doaud-El Baba, and M. Fussenegger, Angew. Chem. Int. Ed., 54, 20, 5933–5938 (2015).
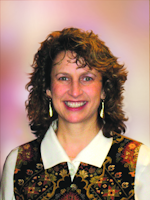
Barbara Gefvert | Editor-in-Chief, BioOptics World (2008-2020)
Barbara G. Gefvert has been a science and technology editor and writer since 1987, and served as editor in chief on multiple publications, including Sensors magazine for nearly a decade.