Photobiomodulation: Regeneration without risk: Light-enabled tissue repair
Affecting biological change by safely exposing endogenous compounds to light is exciting for many reasons. Not only has research demonstrated the effectiveness for such noninvasive therapies, but the approaches are also low cost, thereby promising to allow treatment for individuals across the globe who traditionally have not had access to healthcare technologies.
Often called low-level light therapy (LLLT), photobiomodulation (PBM) therapy is a non-thermal process that uses non-ionizing light sources (either the coherent light of lasers or the non-coherent energy of LEDs) to trigger redox biology-chemical reactions in which the oxidation state of atoms is changed, normally through the transfer of electrons between chemical species.
PBM is being applied and investigated for a wide range of applications, including the harnessing of stem cells for tissue regeneration. Regulatory proteins called growth factors can trigger stem cells (which occur naturally in the adult body) to differentiate into a range of functional cell types. The standard method of boosting stem cell proliferation is a multi-step process that involves extracting tissue, isolating stem cells and processing them in a lab, and then returning them to the body. Recent research shows, however, that noninvasive application of light can boost the natural growth of an individual's own stem cells to enable exciting new treatments.1
Stimulating organ regeneration
When you think of organ regeneration, you may naturally think of the liver. In mammals, this organ has an inherent capacity to recover following injury—an abnormal loss of cells triggers a rapid response.
Among the studies researching the use of light to trigger for regeneration is work by Israeli scientists investigating PBM for liver tissues in the critical 48 hours following injury.2Researchers at Tel Aviv University and Wolfson Medical Center (Holon, Israel) built on earlier work that demonstrated 1) the ability of bone marrow-derived mesenchymal stem cells (MSCs) to naturally stimulate the formation of blood cell components in the liver, and 2) the biostimulatory effects of laser light on cells and organs—including skeletal muscle, heart, brain, and liver following injury.
Led by Uri Oron, Ph.D., at Tel Aviv University's Department of Zoology, the team excised 70% of the livers of 12 mature male rats, which they assigned randomly to two groups: a control non-laser-treated group and an experimental group. The experimental group was treated with 804 nm light from a tunable-power gallium aluminum arsenide (GaAlAs) diode laser with a 1.5-mm-diameter metal-backed glass fiber optic. The researchers applied a 2-cm-diameter beam with 5 mW/cm2 power by placing the distal tip of the fiber optic to a shaved area above the surgical site 3 hours post-surgery for 60 seconds. Two days post-hepatectomy, the researchers injected both groups with 5-Bromo-2'deoxyuridine (BrdU), a synthetic nucleoside used to detect proliferating cells in living tissues.
Examining histological sections from each liver, they found a 2.6-fold increase in the number of proliferating (BrdU-positive) cells per area in the regenerating regions of livers in the laser-treated rats vs. the non-laser-treated group. Likewise, the density of newly formed blood vessels was 3.3X greater and the population of immunopositive stem cells was 2.3-fold higher, in the same area of the laser-treated livers vs. those not receiving laser treatment. The results led the researchers to conclude that PBM was helpful not only in facilitating the formation of new hepatocytes and MSCs, but also for angiogenesis.
For their next investigation, the researchers chose a more-challenging target—the mammalian heart.2 Unlike the liver, the heart's post-injury regenerative capacity is gated by low-level cardiomyocyte proliferation and a limited number of cells expressing stem cell marker proteins. The team tested the hypothesis that applying PBM therapy to MSCs in bone marrow at the tibia would allow the MSCs to migrate to the heart tissue damaged by myocardial infarction (MI; that is, heart attack), and thus reduce the scarring that typically occurs and interferes with normal cardiac functioning.3
The researchers exposed 200 rats to experimental MI. Sham-operated rats served as control, while the rest received light therapy (the researchers applied a GaAlAs diode laser, with power density of 10 mW/cm2, for 100 seconds) to the bone marrow of the exposed tibia at different time intervals post-MI. At three weeks out, the researchers found that rats given laser treatment 20 minutes post-MI had significantly better outcomes compared to the untreated controls. The treated rats' infarct size was diminished 76%, ventricular dilatation was reduced 75%, and there was a 25-fold increase in cell density of MSCs in the infarcted area.
The study demonstrated a novel approach-that of applying PBM therapy to the bone marrow of infarcted rats to trigger production of the rats' own (autologous) MSCs that their systems consequently recruit to the ischemic heart. The use of autologous stem cells as therapeutic agents avoids the cumbersome practice of isolating millions of stem cells, growing them in vitro, and injecting them into subjects.
The study suggests clinical potential for noninvasive (or invasive, using optical fiber in the case of obese individuals) treatment of a patient's bone marrow up to 4 hours post-MI. They hope, too, that the approach can be applied to other organs damaged by ischemia or injury, or undergoing degenerative processes (i.e., neurodegenerative diseases).
Toothsome: Root canal replacement?
How would you like to be able to regenerate damaged teeth rather than having them drilled, filled, or replaced?
Another area of promise focuses on triggering human dental stem cells to form dentin, the calcified tissue that lies just under the enamel in teeth (see Fig. 1). Work in this area earned Praveen Arany, DDS, Ph.D., the 2016 Dr. Horace Furumoto Innovations Young Investigator Award from the American Society for Laser Medicine and Surgery. Now assistant professor of oral biology at the University of Buffalo's School of Dental Medicine, Arany began this effort as a Harvard graduate student and postdoctoral fellow, and continued it while serving as Assistant Clinical Investigator at the National Institutes of Dental and Craniofacial Research (NIDCR) at the National Institutes of Health (NIH).
Under the direction of Wyss Institute Core Faculty member David Mooney, Ph.D., Arany experimented with rodents, drilling holes in their molars and treating the stem cell-rich tooth pulp with low doses of continuous-wave 810 nm light from a GaAlAs laser diode using a 400 μm fiber-optic delivery system.4 Then, he applied temporary caps. After about 12 weeks, high-resolution imaging showed enhanced dentin formation in the animals that had received treatment.
The ability to induce dentin formation in humans would essentially prevent the need for root canal treatments, Arany says, explaining the application of light in deep carious lesions close to the pulp. He also said that using light to generate "an intrinsic dentinal barrier" would free people with sensitive teeth from a lifetime of using desensitizing toothpaste.
Translating these applications to the clinic should be simple, Arany says. While there is plenty of opportunity for development of new devices, currently some available lasers can be applied just by adjusting their wavelength and power settings. So, he has been working to move the applications to human clinical trials.
Endogenous optogenetics?
Unfortunately, though, Arany hasn't yet found much resonance. In fact, people have tended to confuse PBM with optogenetics, which has captured the attention of decision-makers by revolutionizing neurobiology and enhancing the abilities of cell biologists.
A key component of optogenetics is the introduction of exogenously engineered light complexes. Scientists genetically modify target cells to express light-sensitive proteins so that they can control the cell processes precisely and noninvasively. Since its inception, observers have speculated on the possibility of applying optogenetics clinically. After all, photodynamic therapy (PDT) uses exogenous agents to enable light-based therapy. But PDT agents are applied topically—whereupon bodily processes convert them to light-sensitive complexes that respond to the application of light by destroying microbes or tumor cells. This process inherently exhausts the complex, eliminating it from the body.
On the other hand, optogenetics involves irreversible (as far as we know) modifications. So although optogenetics is a tremendous tool for research, its potential as a clinical tool will likely be limited by the inherent difficulty of achieving approval for use of engineered complexes in humans. That said, human trials are anticipated for sight restoration in 2018.5
Because genetic engineering is a key component of optogenetics, the term "endogenous optogenetics" is a bit of an oxymoron. But it's a term people have begun to use because it effectively and appropriately inspires the imagination. The more accurate term for affecting change by targeting endogenous light-sensitive compounds is, of course, PBM.
How does light grow dentin?
To understand the molecular mechanism responsible for laser treatments' regenerative effects, Arany and his colleagues performed culture-based experiments. This work revealed that a naturally occurring cell protein, transforming growth factor beta-1 (TGF-ß1), is critical for triggering the dental stem cells to grow into dentin.
Low doses of laser light induce reactive oxygen species (ROS)—chemically reactive molecules containing oxygen that affect cellular function. The ROS activates latent TGF-ß1, which in turn differentiates the dental stem cells into dentin. His lab is actively exploring these mechanisms with a broader range of adult stem cells—such as those in our bone marrow, skin, and mucosal epithelial stem cells, brain, and eye—to promote directed differentiation to specified tissue.
Understanding for progress
Advancements in PBM haven't necessarily followed the typical progression from lab studies to animal studies, to clinical studies, and then to clinical therapy because of frequently appreciable patient benefits and the complexity of the underlying mechanisms (see Fig. 2). Drawing a parallel to the use of aspirin, Arany explains that while humans have been using it for more than a century, we have only recently discovered some principles of operation. That is why he and his colleagues performed culture-based experiments to uncover PBM's principles of operation (see "How does light grow dentin?").As immediate past-president of the North American Association for Photobiomodulation Therapy (NAALT) and current president-elect of the World Association of Laser Therapy (WALT), groups devoted to promoting low-dose biophotonic treatments, Arany is working to clarify PBM so that misconceptions and terminology don't continue to get in the way of safe, effective, low-cost, noninvasive treatment.
REFERENCES
1. P. R. Arany, Photmed. Laser Surg., 34, 11, 495-496 (2016).
2. U. Oron, L. Maltz, H. Tuby, V. Sorin, and A. Czerniak, Photomed. Laser Surg., 28, 5, 675-678 (2010); doi:10.1089/pho.2009.2756.
3. H. Tuby, L. Maltz, and U. Oron, Lasers Surg. Med., 43, 401-409 (2011).
4. P. R. Arany et al., Sci. Transl. Med., 6, 238, 238ra69 (2014).
5. S. Williams, The Scientist (Nov. 16, 2017); see https://goo.gl/LBN253.
About the Author
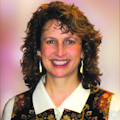
Barbara Gefvert
Editor-in-Chief, BioOptics World (2008-2020)
Barbara G. Gefvert has been a science and technology editor and writer since 1987, and served as editor in chief on multiple publications, including Sensors magazine for nearly a decade.