Raman Spectroscopy: Spectroscopy system outperforms MRI for invasive cancer detection
The dividing line between healthy and cancerous tissues is better characterized as a gray area. In diffusely invasive brain cancers, for instance, diseased cells are tightly packed in the center of the main tumor mass, and extend out in a decreasing gradient.
Surgical removal of tumors is the best treatment, but current methods do not address the invasion of cancer cells into healthy tissue. And until now, no technology has proven effective for detecting invasive cancer.2 Existing clinical technologies—including state-of-the-art neurosurgical microscopy and magnetic resonance imaging (MRI, used either pre- or intra-operatively)—cannot detect the full extent of cancer invasion. Even protoporphyrin IX (PpIX) and 5-aminolevulinic acid (5-ALA)-based fluorescence-guided surgery has limitations for detecting grade 2 gliomas and associated cancer cells.
Likewise, because no adjuvant therapies (such as radiation and chemotherapy) have proven effective for such brain cancers, the inability to fully visualize invasive cells has a pronounced affect, as incomplete resections mean increased mortality among patients. More than 85% of glioblastoma (GBM) recurrences are based in the cavity margin and when it comes to brain surgery, excising more tissue than is absolutely necessary has decided risk as well. Further, the standard of care in surgical treatment of brain surgery—unlike cancers of the skin, breast, mouth, throat, and colon—does not call for multiple tissue biopsies to assess tumor margins.
For these reasons, a great need exists for practical tools that fit into the current surgical workflow, and that enable surgeons to visualize cancer in real time as they operate.
Technology approaches
Among the techniques advanced to surpass MRI and visual assessment of cancer is fluoro-ethyl-tyrosine positron emission tomography (PET). Used for surgical planning, it has demonstrated sensitivity for detecting grade 3 and 4 gliomas (88%), but just 54% for lower-grade gliomas. Other techniques such as ultrasound and optical coherence tomography that provide structural information in real time, and 5-ALA fluorescence-based intraoperative confocal microscopy, have different limitations.
Raman spectroscopy, which provides spectral information based on the vibrational and rotational modes of molecular species in tissue, offers potential for overcoming these limitations. Raman spectroscopy leverages the inelastic scattering of incident near-infrared (near-IR) laser light to enable noninvasive, in vivo analysis of tissue: The spectra it produces enable not only identification of different molecular species, but also determination of their relative concentration.
Since 2013, Canadian researchers Kevin Petrecca of McGill University and Frédéric Leblond of Polytechnique Montreal have led development of a Raman-based instrument. Studies that examined the effectiveness of their handheld probe in measuring normal, cancerous, and necrotic brain tissues demonstrated that real-time inelastic scattering measurements can distinguish necrosis from vital tissue (including tumor and normal brain tissue) with great accuracy, sensitivity, and specificity.1,2 More recently, the team demonstrated that noninvasive, label-free Raman spectroscopy can detect invasive cancer cells well beyond cancer detected on MRI in humans during surgery—up to ~3.7 cm and ~2.4 cm beyond T1-contrast enhanced and T2 MRI boundary, respectively.5
Spectroscopic system development
The real-time in vivo neurosurgical system that they developed comprises a handheld fiber-optic probe (with seven fibers dedicated to detection, and a single, central fiber used for excitation), a 785 nm excitation laser, and a high-resolution CCD spectroscopic detector. In 2015, they demonstrated Raman spectroscopy's ability to detect gliomas of all grades and cancerous invasion of tissue (including previously undetectable cancer diffusely invading healthy tissue, at cellular resolution) with greater than 90% accuracy in 17 patients.
But Raman spectroscopy is associated with low signal-to-noise ratio (SNR), and the glioma study revealed the extent to which artefacts, introduced by various light sources in the operating room, corrupted the inelastic scattering spectra and rendered the system's measurements useless for quantitative assessment. Because requiring surgeons to turn off lights to use their system would hamper its acceptance, the team began efforts to overcome the artefact problem.
To boost the Raman signal, they deployed supervised machine learning with artificial neural networks (ANNs). ANNs use a nonparametric model, suited for handling the inconsistent effects of light artefacts on the Raman spectra, that can adapt to nonlinear changes in optical signal.3 They used ANN classification to compensate for sources that included overhead lights in the operating theater and IR light from a neuronavigation system.
In addition, they integrated a custom optical filter system with the front lens of the neurosurgical microscope to allow in vivo Raman measurements in the presence of the intense microscope light.4 A machine learning technique demonstrated that the interference filters attenuated the arc lamps' near-IR peaks to the point of allowing differentiation between white and gray matter at 100% accuracy. Without the filter, residual light artefacts produced a 33% drop in specificity.
The most recent development of the system has been augmentation of the Raman approach with two additional spectroscopy modes: intrinsic fluorescence spectroscopy (IFS, which detects such endogenous fluorophores as enzymes, metabolic cofactors, amino acids, porphyrins, and structural proteins) and diffuse reflectance spectroscopy (DRS, which provides information from such tissue absorbers as oxygenated and deoxygenated hemoglobin, lipids, water, cytochromes, and melanin).6 IFS had previously proven effective in detecting bronchopulmonary, laryngeal and breast cancers, as well as epithelial precancerous tissue, while DRS has been shown to detect cervical, breast, and skin cancers (both melanoma and nonmelanoma), as well as colorectal metastases to the liver. The researchers hypothesized that IFS and DRS could complement Raman spectroscopy synergistically, and thereby maximize cancer detection. They tested their hypothesis by designing an intraoperative, label-free, optical system that integrates the three approaches to boost sensitivity to nearly 100%.
The combination approach exploits nonredundant biomarkers (including molecular species with specific signatures for fluorescence, absorption, and molecular vibration), and uses a hierarchical supervised machine learning algorithm to optimize the diagnostic potential of all three methods.
System, components, and operation
The team's latest design acquires Raman and IFS spectra sequentially for two excitation wavelengths, and DRS spectra based on excitation with a broadband white-light source (see figure). Application software designed using National Instruments' LabVIEW controls the light sources, filter wheel, and detectors, and a 3 m fiber-optic cable connects the probe to the illumination/detection subassembly. Within the cable, a central 272 mm core silica fiber (which provides Raman spectroscopy tissue excitation at 785 nm) is surrounded by nine low hydroxyl-content 300 mm core silica fibers, seven of which collect Raman-scattered light—the other two are dedicated to IFS and DRS.
The re-emitted signal passes through a notch filter, which removes elastically scattered light. An in-line short-pass emission filter, placed in front of the excitation fiber, helps minimize the impact of the Raman signal from the optical fibers. A custom two-component lens at the tip of the probe ensures excitation. Detection areas for all fibers exactly overlap, thus making Raman spectroscopy, IFS, and DRS measurements spatially colocated with a 0.5 mm interrogation area. A single optical connector maximizes SNR by combining all seven Raman spectroscopy fibers—for the IFS/DRS fibers, one connector handles excitation while another covers detection.
The Raman spectroscopy detection connector links to a spectrograph incorporating a diffraction grating and a high-resolution CCD camera. Excitation is provided by a single-watt, 785 nm spectrum-stabilized near-IR laser with adjustable power (for Raman spectroscopy), two LEDs (365 and 455 nm, for IFS), and a 3 W 470–850 nm broadband LED (for DRS). A low-pass filter that sits between the IFS sources and the probe's excitation fiber serves to minimize light bleedthrough into the detection fiber, and an optical switch manages excitation among the IFS and DRS sources, sequentially.
A compact spectrometer provides IFS and DRS detection, and a motorized, five-slot filter wheel sits between it and the detection fiber. On the IFS channels, a long-pass filter restricts the quantity of excitation light arriving at the spectrometer. The custom software orchestrates acquisition using the various modalities (Raman spectroscopy, IFS, and DRS).
Clinical application and commercialization
Using this multimodal optical molecular imaging system, the team proved able to detect brain, lung, colon, and skin cancers in situ, during surgery to with astonishing performance: 97% accuracy, a full 100% sensitivity, and 93% specificity. The approach promises to profoundly impact a wide range of oncology interventions, both surgical and noninvasive.
In 2015, the group formed a company (ODS Medical, www.odsmed.com) to commercialize it. Thus far, the company has raised first-seed funding and achieved admission into the U.S. Food and Drug Administration's (FDA) expedited access program (EPA). In June 2017, the company launched a randomized controlled trial at the Montreal Neurological Institute and Hospital.
The designers researched such factors as signal linearity with integration time and laser power and their impact on SNR, and optimal data acquisition parameters. Their resulting handheld contact probe is promising for live, local detection of cancer cells in the human brain, including intraoperative glioma resection. It has a small footprint, fast acquisition time, and is highly accurate. It can detect invasive cancer cells without disrupting the workflow, and could complement or even replace MRI as a technique to identify cancer margins.
REFERENCES
1. J. Desroches et al., Biomed. Opt. Express, 6 7, 2380–2397 (2015).
2. M. Jermyn et al., Sci. Transl. Med., 7, 274ra19 (2015).
3. M. Jermyn et al., J. Biomed. Opt., 21, 9, 094002 (2016).
4. J. Desroches et al., Analyst, 142, 1185–1191 (2017).
5. M. Jermyn et al., Biomed. Opt. Express, 7, 12, 5129–5137 (2016).
6. M. Jermyn et al., Cancer Res., 77, 14, 1–9 (2017).
About the Author
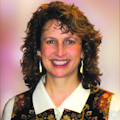
Barbara Gefvert
Editor-in-Chief, BioOptics World (2008-2020)
Barbara G. Gefvert has been a science and technology editor and writer since 1987, and served as editor in chief on multiple publications, including Sensors magazine for nearly a decade.