Advanced Microscopy: Adaptive optics open a new frontier of in vivo subcellular imaging
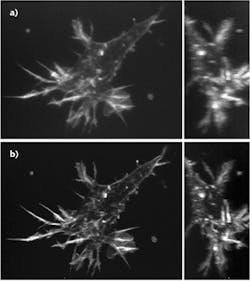
"Adaptive optics will soon be an essential element for all high-resolution imaging deep in multicellular specimens," concludes a newly published review by Na Ji.1 Formerly Group Leader at Janelia Research Campus, Howard Hughes Medical Institute (Ashburn, VA; where she worked with Nobel Laureate Eric Betzig), and now Associate Professor of Physics and Neurobiology at her PhD alma mater, University of California at Berkeley (which has also welcomed Betzig to its staff), Ji develops imaging technology for application to neurobiology—most interestingly, perhaps, in vivo brain research.
Betzig, of course, shared receipt of the 2014 Nobel Prize in chemistry for development of super-resolved fluorescence microscopy, a technique that allows subcellular imaging of biological specimens ex vivo. But putting cells on a microscope slide doesn't allow understanding of the full context of their existence in a living organism. That's why he and Ji, among others, are looking to enable subcellular imaging deep into the tissues of living, behaving animals.
Why adaptive optics?
The resolution of an optical system is theoretically constrained only by the diffraction of light waves. However, optical effects induced by the specimen (or the optical system itself) result in blur. Refractive index varies throughout three-dimensional (3D) tissues, decreasing both contrast and signal with increased structure complexity and depth.
As described in the 2013 book Adaptive Optics for Biological Imaging,2 adaptive optics (AO) is the most powerful and versatile approach researchers have developed to correct these aberrations. AO systems are designed to actively control and compensate for degradation and thus sharpen imagery.
Originally developed for astronomy, where it has taken more than 60 years to become mainstream, AO has been applied in biological imaging—first to retinal imaging and then microscopy—since the late 1970s.
Traditional AO microscopy systems correct the wavefront by addressing the signal received at a photodetector instead of attempting to measure the wavefront directly. That's because adding a wavefront sensor to an optical system is a complex matter, and because no natural point-source reference (such as the "guide star" used for AO in astronomy) exists in biology. But in the past 15 years or so, researchers have developed a sensorless AO, and investigated implementation in two-photon microscopy.
Recent advances drive neuroscience
Ji, Betzig, and colleagues note that optical imaging is facilitating more ambitious neuroscience research.3 Included in such work is a goal to understand the central nervous system's functional architecture, as well as communication dynamics of various neuron types and their impact on behavior—achieving this goal requires the ability to observe large populations of neurons in vivo at single-synapse resolution. They developed an approach that reliably produces morphological and functional imaging with diffraction-limited quality at a depth of 700 μm within the brain of a living mouse.4
It uses two-photon excitation to create a laser-induced near-infrared (for deep penetration) guide star and a Shack-Hartmann wavefront sensor (SHWS) to measure the guide star's wavefront distortion using an array of lenslets, each of which samples a portion of the incidence wavefront and focuses light on an imaging sensor. A wavefront-modulating element then compensates for the aberrations. This compensation normally involves software algorithms triggering actuators to deflect the surface of a deformable mirror, allowing light to exit the mirror in parallel planes.
A number of other publications in 2017 have demonstrated progress in AO microscopy for neuroscience. For instance, French researchers have recently demonstrated a theoretically optimized wavefront correction method for inhomogeneously labeled biological samples at a depth of 200 μm in brain tissue.5 And researchers at the National Institute of Biomedical Imaging and Bioengineering (NIBIB; Bethesda, MD) created an instant structured illumination microscopy (ISIM)-based system that likewise uses two-photon excitation to create a guide star to enable distortion measurement—which provides the basis for the actions of a deformable mirror (see Fig. 2).6 Their work is focused on high-speed generation of aberration-corrected images. "The faster we can image live samples and the less we can interfere with their environment, the better we can understand how biology truly works," said team leader Hari Shroff, Senior Investigator at NIBIB's Section on High Resolution Optical Imaging (HROI).
Imaging larger areas
Conventional adaptive optics systems are limited because they can image only small areas at a time, but a team at Purdue University (West Lafayette, IN) led by Meng Cui has developed Multi-Pupil Adaptive Optics (MPAO), which enables simultaneous wavefront correction over a large field of view (see Fig. 1).7 In the current implementation, MPAO increases the correction area by approximately an order of magnitude, and allows up to 32 MHz voxel rate at the limit of the laser scanning hardware. The initial research used a 3 × 3 prism array containing a total of nine segments, each about 1 cm2. Now, the team is working to improve performance by increasing the size of these arrays to contain perhaps as many as 36 segments.MPAO's capability of independent wavefront control also enables high-speed nonplanar imaging of 3D dynamics. The researchers have applied MPAO to in vivo dynamic imaging of microglia and blood vasculature, and structural and calcium imaging of neuronal network in mammalian brain.
"We are looking at huge numbers of neurons, so the number of data points you can measure per second is 20 million, 30 million," said Cui, assistant professor of Electrical and Computer Engineering and Biological Sciences. "High throughput is very important because you want to measure these numerous neurons simultaneously at very high speed and also at high spatial resolution."
Along similar lines, researchers at the University of California, Santa Cruz (UCSC) have demonstrated large-volume wavefront shaping through a scattering layer with a single correction.8 Their technique, called conjugate adaptive optics and remote focusing (CAORF), provides a wider correction volume. In tests, their proposed system provided 10X wider axial field of view compared with a conventional conjugate AO system where 16,384 segments are used on a spatial light modulator.
Incidentally, UCSC is headquarters for the Center for Adaptive Optics (CfAO), a Science and Technology Center that was funded for 10 years by the National Science Foundation (NSF; Arlington, VA) and is now funded by the University of California (UC). Members include researchers from eight other UC campuses, and the center has many academic and industrial affiliates. Its aim is to advance and disseminate AO for science, health care, industry, and education, and to lead by developing and demonstrating improvements in AO, and catalyzing advances.
The CfAO holds a Summer School program each year to teach graduate students methods of inquiry-based science teaching, and to apply this knowledge in programs that attract and retain a new generation of scientists, particularly women and underrepresented minorities. Sammy Weiser Novack presents a section on AO for biological imaging that reviews the principles of AO in the context of biological microscopy. The 2017 edition will focus on applications developed in collaboration with Joel Kubby's lab at UCSC, and applications from other labs.
Toward new insight
As Ji explains, AO microscopy research has thus far focused on development of technological approaches. The next steps, now underway, are to hone these methods for improved performance and ease of use, and ultimately to leverage them to enable biological discovery. A recent in vivo imaging study by her team at Janelia Research Campus is the first we know of to use AO to enable new biological insight-in this case, regarding central nervous system functioning.9
REFERENCES
1. 1. N. Ji, Nat. Methods, 14, 374–380 (2017).
2. J. A. Kubby (ed.), Adaptive Optics for Biological Imaging, CRC Press (Taylor & Francis Group), Boca Raton, FL; ISBN: 978-1-4398-5018-3 (2013).
3. K. Wang, W. Sun, N. Ji, and E. Betzig, "In vivo brain imaging with adaptive optical microscope," Proc. CLEO 2016, paper Am4O.1 (2016); https://doi.org/10.1364/cleo_at.2016.am4o.1.
4. K. Wang et al., Nat. Commun., 6, 7276 (2015); doi:10.1038/ncomms8276.
5. D. Champelovier et al., Sci. Rep., 7, 42924 (2017).
6. W. Zheng et al., Nat. Methods (2017); doi:10.1038/nmeth.4337.
7. J.-H. Park, L. Kong, Y. Zhou, and M. Cui, Nat. Methods, 14, 581–583 (2017); doi:10.1038/nmeth.4290.
8. X. Tao et al., Opt. Express, 25, 9, 10368–10383 (2017); doi:10.1364/oe.25.010368.
9. W. Sun, Z. Tan, B. D. Mensh, and N. Ji, Nat. Neurosci., 19, 308–315 (2016).
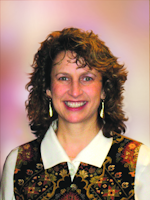
Barbara Gefvert | Editor-in-Chief, BioOptics World (2008-2020)
Barbara G. Gefvert has been a science and technology editor and writer since 1987, and served as editor in chief on multiple publications, including Sensors magazine for nearly a decade.