OPTOELECTRONIC THEORY: Optical capacitor leverages light’s magnetic field
Imagine converting the Sun’s energy to electricity without the need for expensive semiconductor materials or the usual absorption-exciton-drift-current conversion steps. This is precisely what researchers at the University of Michigan (Ann Arbor, MI) are doing: developing a capacitive energy source, or “optical capacitor,” based on their discovery that the heretofore-considered weak magnetic component of light can—under the right conditions—be exploited to generate an intense magnetoelectric effect.1 Not only is efficient optical charge separation possible, but the effect can also produce magnetically induced terahertz radiation.
The right conditions
The better known but weak inverse Faraday effect produces a small, quasi-static magnetic field along the direction of propagation of light (perpendicular to the magnetic component of its electromagnetic field) when the light is circularly polarized. But when linearly polarized light of an intensity as low as 107 W/cm2 travels in certain transparent dielectrics, charge oscillations driven by the electric and weak magnetic component of the light can drive a dipolar magnetization parallel to the optical magnetic field in bound electron systems, creating intense magnetic dipole radiation—a magnetoelectric effect a million times more intense than the weak inverse Faraday effect.
In this magnetoelectric interaction, each illuminated atom or molecule in the dielectric medium acquires a large static electric dipole moment—a static polarization that is sustained by a complex sequence of events: 1) the electric field initiates electron motion parallel to the electric field; 2) the magnetic field component causes a deflection of the electron (from the Lorentz force) that grows in amplitude due to a coupling between electric and magnetic-field driven motions (analogous to the coupled mechanical vibrations that destroyed the Tacoma Narrows Bridge in Washington State in 1940); and 3) the average position of the electron shifts away from the nuclei of the surrounding atoms and molecules, effectively accomplishing charge separation and creating an optically charged capacitor that could be used as an electrical energy source.
This magnetoelectric effect is quantified by the researchers through a classical Lorentz oscillator model (see figure). When electron motion is compared using low-intensity light incident on the dielectric (1 V/m) versus high-intensity light (108 V/m), the electron motion switches from being along the electric field (x) as expected to having a large component along the direction of motion of the light beam (z). At higher light intensities, this magnetically driven response attains its maximum amplitude with respect to the linear electric polarization of the dielectric medium, and the electron motion is sustained in a curved path beyond the nucleus. By applying magnetic-susceptibility equations, the surface-charge density possible in a plane-parallel dielectric slab illuminated by a known intensity of coherent light can be calculated.
If ultrashort pulses are used as the illumination source, an additional capability beyond charge separation is possible: the generation of terahertz radiation. When any electric dipole induced by light is short-lived (transient), it becomes an antenna. If the inducing pulses are short enough, the frequency of the radiation emitted by the antenna has a breadth Δν, as determined by the uncertainty principle (Δν is approximately 1/Δt), that can extend to the terahertz range.
Optical-to-electrical conversion
In theory, if a 1 kW, 800 nm Gaussian source with a pulse-repetition rate of 25 MHz is focused to a spot size of 50 µm in a 10-m-long sapphire optical fiber, the calculated optical-to-electrical conversion efficiency of the magnetoelectric system would be around 30%; additional calculations show that ordinary sunlight focused into a large-core optical fiber through concentrating optics could result in 10% conversion efficiency values, despite the incoherent nature of sunlight. While the physical experiment has not yet been completed, the researchers are confident of the result.
“Since the light is not absorbed during the magnetoelectric interaction in transparent conversion media, much less heat is expected than in photovoltaic technology and light will simply pass through the converter when electrical power is not actively being drawn from it,” says professor Stephen Rand at the University of Michigan. “This is potentially more eco-friendly. Any light not directly converted to electricity could be used for growing crops or passed through to solar cells to boost the overall conversion efficiency of hybrid systems. Magnetoelectric technology is also an interesting contender for more efficient, transportable power generation in beamed laser-power applications or for solar conversion in harsh environments like space, where semiconductor circuits degrade more rapidly than radiation-hard dielectric materials.”
REFERENCE
1. W.M. Fisher and S.C. Rand, J. Appl. Phys., 109, 064903 (2011).
About the Author
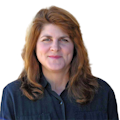
Gail Overton
Senior Editor (2004-2020)
Gail has more than 30 years of engineering, marketing, product management, and editorial experience in the photonics and optical communications industry. Before joining the staff at Laser Focus World in 2004, she held many product management and product marketing roles in the fiber-optics industry, most notably at Hughes (El Segundo, CA), GTE Labs (Waltham, MA), Corning (Corning, NY), Photon Kinetics (Beaverton, OR), and Newport Corporation (Irvine, CA). During her marketing career, Gail published articles in WDM Solutions and Sensors magazine and traveled internationally to conduct product and sales training. Gail received her BS degree in physics, with an emphasis in optics, from San Diego State University in San Diego, CA in May 1986.