PHOTONIC FRONTIERS: PETAWATT LASERS - A proliferation of petawatt lasers
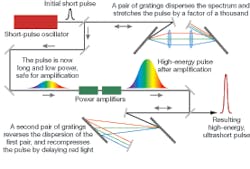
Squeezing energetic laser pulses down to ultrashort durations can generate tremendous peak powers. A decade ago the Lawrence Livermore National Laboratory (LLNL; Livermore, CA) blazed the trail by modifying one arm of its Nova fusion laser to create the Petawatt Laser, which delivered pulses exceeding 1015 W (1 PW). Now some 20 petawatt lasers are in operation or development around the world, and European planners are aiming for an exowatt (1018 W) laser (see table).
Some major petawatt laser projects
table
meters
Extreme_Light_
Infrastructure/
EliWeb1.html
rochester.
edu/index.php
str/MPerry.html
forschung/
phelix
optics.de/
ultraphotonics/
polaris_
laser.html
edu/~utlasers/
petawatt_files/
petawatt.htm
gov/JLF/jlf.html
uk/Facilities/
vulcan/
index.htm
sandia.gov/
petawatt/p_
program.html
Focusing petawatt pulses onto small spots can produce extreme power densities, opening new domains in light-matter interactions. At 1018 W/cm2 electrons are accelerated to relativistic speeds, doubling their mass. Such high power densities also can generate x-rays and gamma rays, produce high-order harmonics, and accelerate electrons and protons. Petawatt lasers have since pushed power densities to the 1021 W/cm2 range. Europe’s planned “Extreme Light Infrastructure” will reach power densities to 1023 W/cm2, entering the unexplored “ultrarelativistic” regime, in which acceleration increases electron mass by a factor of 10,000 and particles can be produced as well as photons. It promises a whole new world of laser-matter interaction.
Chirped-pulse amplification
Peak focused intensity available from lasers or amplifiers stalled out in the 1014 W/cm2 range until the mid 1980s, limited by nonlinear effects and optical damage. Chirped-pulse amplification got around those problems by stretching the pulse duration before it reached the amplifier, keeping peak power at safe levels, and then compressing the output pulse to the original length. The trick relied on passing broadband light from a pulsed oscillator through highly dispersive optical gratings before delivering them to the amplifier. This procedure delayed the light as a function of wavelength, stretching out the pulse to limit the instantaneous peak power in the amplifier. A second grating then compensated for dispersion, squeezing the extended pulses back into ultrashort pulses (see Fig. 1). This allowed the use of solid-state media such as neodymium-doped glass and titanium-doped sapphire, which can store about a thousand times more energy than the dye or excimer lasers used earlier.
The oscillator must be broadband to generate an ultrashort pulse, but there are tradeoffs in selecting the amplifier. Neodymium-doped glass can be made in large volumes and can generate high-energy pulses, but its bandwidth is limited; pulse energies can exceed hundreds of joules, but pulse durations typically are at least 500 fs. Titanium-doped sapphire offers much higher bandwidths, but requires a laser pump and the crystal size is limited-which restricts pulse energy to a few joules, but allows pulse lengths as short as 20 fs. This dichotomy creates two distinct petawatt pulse regimes-high energy from Nd:glass and short pulse from Ti:sapphire.
Intermediate pulse lengths and powers can be produced by optical parametric chirped-pulse amplification followed by a glass amplifier. In this case, pulses from a Ti:sapphire oscillator are chirped and mixed with light from a high-power doubled-neodymium pump in a nonlinear crystal such as beta barium borate. Optical parametric amplification transfers energy from the pump beam across the whole bandwidth of the Ti:sapphire pulse, and will amplify oscillator pulses to one joule in the Texas Petawatt Laser, says Todd Ditmire of the University of Texas at Austin. Glass amplifiers will provide a final boost to generate 250 J, 150 fs pulses.
The first petawatt laser
Livermore’s Petawatt Laser used a chain of Nd:glass lasers from one beam of the Nova fusion laser to amplify nanosecond pulses to the kilojoule range. Pulses were expanded and compressed with high-efficiency 75 cm gratings. Amplifier output of 1.3 kJ in an 800 ps pulse could be compressed down to a 430 fs pulse with peak power of 1.3 PW, which in turn could produce power density approaching 1021 W/cm2. The system generated its first petawatt pulse on May 23, 1996, and ran for three years until Nova was dissembled in 1999.
The Livermore experiments demonstrated the potential of petawatt lasers to concentrate tremendous energies into small volumes, opening a new regime of high-temperature and high-pressure matter for study. The intense fields could accelerate both electrons and positive ions to high velocities over short distances (see www.laserfocusworld.com/articles/252490). Experiments generated bright beams of high-energy x-rays and gamma rays. And Livermore also showed that firing petawatt lasers into a laser-heated fusion target produced a powerful shock wave that helped ignite the fusion fuel.Second-generation petawatt lasers
The second generation of petawatt lasers is already operating. The Rutherford Appleton Laboratory (Didcot, England) uses a Ti:sapphire oscillator and an optical parametric amplifier to preamplify pulses which then pass through a beam of the lab’s Vulcan Nd:glass laser, and three additional 208 mm Nd:glass disks salvaged from Nova (see Fig. 2). Commissioned in 2002, it initially produced 800 fs pulses with peak power of 500 TW. Further refinements ramped up power, which reached the petawatt level in October 2004, delivering 423 J onto the target in a 410 fs pulse.
Livermore has built a second-generation petawatt laser called Titan around the old two-beam Janus Nd:glass laser used in fusion target experiments back in 1975, says Andrew Ng of Lawrence Livermore National Laboratory (LLNL). Overhauled with better glass, the system has two independent beam lines for chirped-pulse amplification and a new generation of pulse-compression gratings. The first experiments in June 2005 generated 400 J in 400 fs to reach petawatt peak power focusable onto an 8 µm spot. It also can operate in long-pulse mode, generating 1 kJ in less than 3 ns or 140 J in 250 ps. Titan can fire long and short pulses simultaneously from its two arms. Ng says that firing long pulses to create a plasma and short pulses to probe the plasma is a very effective way to study high-energy states.
Livermore is also planning a big step up in energy with a second long-pulse system for use with the National Ignition Facility. Called the Advanced Radiographic Capability, it initially will fire 1 kJ pulses to record multiframe x-ray movies of NIF targets, says lead scientist Chris Barty of LLNL. By combining four NIF beams, he hopes to generate 13.2 kJ in a 10 ps pulse. The first beamline is to be commissioned in spring 2009.
Most other systems in operation, construction, or planning stages are either long-pulse systems based on Nd:glass or Ti:sapphire systems generating pulses as short as 20 fs. The main exception is the $15 million Texas Petawatt Laser, which will use parametric amplification to raise the 1 J output of a Ti:sapphire oscillator to 250 J, which they hope to deliver in 150 fs pulses. Project director Todd Ditmire hopes to produce his first petawatt pulses late in 2007.
Extreme Light Infrastructure
The biggest plan in the works is the “Extreme Light Infrastructure” (ELI)-an exowatt laser facility proposed for construction as part of the European Commission’s roadmap for new research infrastructure. It also would generate 10 kJ pulses, but it would compress them to 10 fs, and fire one shot per minute. The five-year construction project would cost €116 million (US$147 million), with annual operating costs and salaries reaching €8 million (US$10 million). The plan has yet to be officially approved, “but it is going to happen,” says Gerard Mourou, director of the Laboratoire d’Optique Appliquée (Palaiseau Cedex, France), which is spearheading the Europe-wide planning group.
Reaching those goals would produce on-target power densities above 1023 W/cm2 in the “ultrarelativistic” region, where acceleration multiplies electron mass by a factor of 10,000 or more. Computer models predict that such power densities should make it possible to produce electron-beam pulses lasting less than 5 fs with gigaelectronvolt energies, and multi-GeV proton beams that could generate pions, muons, and neutrinos. Relativistic compression might produce pulses in the attosecond (10-18 s) or zeptosecond (10-21 s) regimes. The ELI proposal states, “ultrarelativistic intensities could unify nuclear physics, high-energy physics, astrophysics, and cosmology.”
We are not there yet, but petawatt pulses provide a unique tool to probe matter. Charged-particle accelerators push small groups of particles to very high energies, but the numbers are so low that the total energy is not very high so they’re not good for heating matter. “The attraction of lasers is their ability to deposit a lot of energy in a small volume; a little cube of energy, which we put into matter faster than the matter can expand,” says Ditmire. The result is the hottest, densest matter that we know how to generate in the laboratory, a promising new research frontier.
FURTHER READING
G. Mourou et al., Physics Today, Jan. 1998, p. 22.
R.F. Service, Science 301, 154 (2003).
J.D. Zuegel et al, Fusion Science and Technology 49 453, (April 2006).
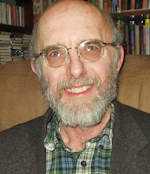
Jeff Hecht | Contributing Editor
Jeff Hecht is a regular contributing editor to Laser Focus World and has been covering the laser industry for 35 years. A prolific book author, Jeff's published works include “Understanding Fiber Optics,” “Understanding Lasers,” “The Laser Guidebook,” and “Beam Weapons: The Next Arms Race.” He also has written books on the histories of lasers and fiber optics, including “City of Light: The Story of Fiber Optics,” and “Beam: The Race to Make the Laser.” Find out more at jeffhecht.com.