Terahertz imaging advances toward medical diagnostics
The field of terahertz (THz) imaging based on time-domain spectroscopy (TDS), a versatile technique for gathering images that enable us to “see through” plastics, textiles, and cardboard, has made enormous progress during the past two decades. Terahertz wavelengths—ranging from approximately 30 µm to 3 mm—are strongly absorbed by liquids and metal material, which makes terahertz imaging ideal for use identifying such materials in applications like airport security scanners and food inspection.
Unlike x-rays, terahertz waves have low photon energies in the range of 0.4 to 40 meV, which is not harmful to biological tissue. The ability of THz-TDS systems to acquire x-ray-like images without dangerous ionizing radiation makes it particularly compelling for their potential in living tissue (in vivo) applications. Terahertz waves are absorbed by water, so they are handy for distinguishing varying water content in different objects. Plus, using the unique spectral fingerprint of many biological organisms and molecules, terahertz can provide spectral information about each pixel within an image, like hyperspectral imaging cubes, which adds to the exciting potential of terahertz technology for medical diagnostics, such as cancer detection and diabetes screening. Furthermore, imaging with terahertz sources can provide sensitivity in soft tissues comparable to that of x-rays.
Commercial, portable passive terahertz systems have been on the market for more than two decades, thanks to the advent of very sensitive detectors, such as fiber pigtailed antennas. Systems using gallium arsenide (GaAs) Schottky diode detectors, superconducting multiple-quantum-well antennas, and planar detector arrays have also found widespread use in a variety of nondestructive, noninvasive applications, including security, food inspection, manufacturing, and art conservation. In recent years, terahertz stand-off detection has been used by major transportation hubs in 18 countries. For example, London’s Underground and the LA Metro use the technology to scan passengers for concealed weapons or other contraband as they proceed through terminals. One such device, the VariView tactical awareness camera, licensed by Thruvision (Abingdon, UK), uses thermal contrast to detect potential weapons on the body, speeding up security screening of passengers (see Fig. 1).1 But these systems don’t gather spectroscopic information, so they aren’t as useful for biomedical applications.
Likewise, the advent of new terahertz sources in recent years has combined with these sensitive detectors to enable the first commercial, portable THz-TDS systems that actively illuminate the target to acquire spectroscopic information.
Typically, to create terahertz waves for TDS, the source is a pulsed, mode-locked fiber laser, often at femtosecond frequencies. Fiber lasers offer compactness that enables portability. Other types of terahertz sources proven in the lab with promise for commercial medical applications include photoconductive antennas, powerful gyrotrons with both continuous-wave (CW) and pulsed modes, and quantum cascade lasers.
Although terahertz imaging has yet to reach commercial viability within the medical field, according to Qiwu Shi, professor of Material Science and Engineering at Sichuan University (Chengdu, China), research in pursuit of in vivo applications has greatly accelerated in recent years.
“Advanced terahertz imaging systems are becoming higher resolution, smaller in size for better portability, and faster in imaging,” says Shi. “Also, the commercial development of numerous advanced terahertz sources has rapidly reduced the cost.”
Barriers
One of the biggest limitations of terahertz is its long wavelengths and weak light-matter interaction, which means it can only penetrate a few hundred microns through live tissue. At 0.5 THz, the penetration depth of fat is only a few millimeters, but only a few hundred microns in fibrous tissue or cancer. The strong absorption of terahertz by water in vivo causes deterioration of the reflected information and the image. Clinicians can get around this problem using external (in vitro) techniques, such as freeze-drying, endoscopy, or studying slices of tissue.2
Another emerging solution to reduce image deterioration in high-water-content tissues in terahertz imaging is the use of contrast agents. Nanoparticle contrast agents have been developed in a technique called terahertz molecular imaging, which increases the reflective index of the terahertz water near the nanoparticles. Gold nanorods (GNRs) are the most commonly used contrast agents in terahertz imaging experiments, thanks to their ability to create surface plasmon resonance absorption in response to near-infrared lasers. The enhanced light absorption can cause photothermal conversion and then lead to increased terahertz reflection amplitude. In live cancer cells enhanced with GNRs, terahertz reflection showed a 20% increase over those without GNRs. Other contrast agents with potential in terahertz medical imaging include superparamagnetic iron oxide nanoparticles and gadolinium oxide nanoparticles.
“The most promising point for terahertz medical imaging is the emergence of contrast agents, but more study is needed on their biotoxicity before they can reach clinics,” says Shi.
Ultimately, in vivo terahertz imaging works well when the area to be imaged is literally (and only barely) skin deep. In Scientific Reports, an interdisciplinary team demonstrated that direct terahertz time-domain imaging can be used as a safe and effective diagnostic test for diabetic foot syndrome.
Physics postdoctoral researcher Goretti Hernandez-Cardoso at the Philipps-Universität Marburg (Germany) and colleagues at the Center of Investigations in Optics, Instituto Mexicano del Seguro Social, and Hospital Angeles Leon (all three in Guanajuato, Mexico) used a terahertz spectrometer from Advanced Photonix (Camarillo, CA; now Luna Innovations, Ann Arbor, MI) coupled to an imaging platform to raster scan and image the soles of healthy vs. diabetic patients. The spectrometer used a Yb:fiber femtosecond-pulsed laser, photoconductive antennas, and a sophisticated algorithm to map hydration levels of the skin. The team analyzed the water content of the patients’ feet to predict deterioration in early stages of the disease, which can help prevent ulcers that can lead to amputation (see Fig. 2).3 This was the largest human population to ever be imaged using terahertz technology. The findings also provided evidence of a link between the presence of neuropathy, common in diabetic foot patients, and the dehydration of their feet, possibly due to the failure of the nervous system to help regulate hydration.How about if the target to be imaged is, say, as wide as a tooth? Could terahertz imaging replace x-rays in the detection of dental caries? A group of scientists at Hubei Polytechnic University and Nanjing University in China set out to show terahertz equipment can do just that. Dr. Nagendra Paradad Yadav and colleagues constructed a terahertz parametric imaging system to examine teeth in 2D and 3D. The team used a Tera-1024 terahertz camera from TeraSense (San Jose, CA), and a fixed-frequency 100 GHz (0.1 THz) pulsed diode at various power settings ranging from 80 mW to 400 mW.4 The setup revealed terahertz imaging can provide rapid diagnostic information on enamel, dentine, and caries. Future studies will work on increasing terahertz imaging resolution to better than 1 mm.
Another advance toward biomedical use of terahertz imaging is machine learning to assist in processing the large amount of digital information obtained via images and spectra. Commercial detector arrays used for terahertz imaging can measure 1024 × 1024 pixels and larger, and if every pixel contains spectroscopic information, the resulting hyperspectral cube is too large for the average non-superhero to analyze efficiently. Pattern recognition algorithms can help identify opaque objects with clear boundaries, a goal for reconstructing 3D terahertz tomographic image cubes both in vivo and in vitro. Machine learning can compare large amounts of spectral information quickly to recognize, for example, cancerous vs. healthy tissue.
A barrier to terahertz in medical applications is the lack of standards. The increasing use of terahertz in dermatology, cosmetology, and communications could increase the incidental exposure of humans to high-energy terahertz sources. Small to moderate periodic doses of terahertz exposure from CW sources cause no damage to live tissue, but lengthy exposure (≥1 hr) to higher-peak-power pulsed terahertz sources, such as those that might be used in cosmetology or cellular phones, could potentially cause DNA damage and changes in gene expression. Even a few seconds of exposure from a high-power terahertz laser can induce thermal injury because intercellular water molecules vibrate within skin. Before in vivo terahertz medical diagnostic equipment can find its way into clinics and labs, more research is necessary to quantitatively define safe and optimal exposure times and dosages.5
“It may be some time before we see commercial terahertz equipment for cancer dental screening,” says Shi, “but it’s exciting to see researchers so focused on the goal.”
REFERENCES
1. K. Hoggan, h4-solutions.com (Oct. 18, 2019), and thruvision.com.
2. Z. Yan et al., TIBTEC online, in press (2022); https://doi.org/10.1016/j.tibtech.2021.12.002.
3. G. G. Hernandez-Cardoso et al., Sci. Rep., 12, 3110 (2022); https://doi.org/10.1038/s41598-022-06996-w.
4. N. Paradad Yadav et al., J. Electron. Sci. and Tech., 19, 3 (2021); https://doi.org/10.1016/j.jnlest.2021.100082.
5. O. Cherkasova et al., J. Biomed. Opt., 26, 9 (2021); https://doi.org/10.1117/1.jbo.26.9.090902.
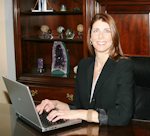
Valerie Coffey-Rosich | Contributing Editor
Valerie Coffey-Rosich is a freelance science and technology writer and editor and a contributing editor for Laser Focus World; she previously served as an Associate Technical Editor (2000-2003) and a Senior Technical Editor (2007-2008) for Laser Focus World.
Valerie holds a BS in physics from the University of Nevada, Reno, and an MA in astronomy from Boston University. She specializes in editing and writing about optics, photonics, astronomy, and physics in academic, reference, and business-to-business publications. In addition to Laser Focus World, her work has appeared online and in print for clients such as the American Institute of Physics, American Heritage Dictionary, BioPhotonics, Encyclopedia Britannica, EuroPhotonics, the Optical Society of America, Photonics Focus, Photonics Spectra, Sky & Telescope, and many others. She is based in Palm Springs, California.