Advances in environmental sensing help improve water quality
Photonics technologies, particularly spectroscopy, are widely used in environmental sensing. Much of our environmental sensing coverage over the past decade has justifiably been centered around standoff detection methodologies, such as how differential optical absorption spectroscopy (DOAS) and differential absorption lidar (DIAL) are applied to measure air pollution. While these techniques are fascinating from a technical perspective and show great potential for reducing air pollution, they represent only a tiny percentage of the overall photonics impact on the field of environmental sensing. In fact, an argument can be made that over the past quarter-century, in situ ultraviolet (UV) spectroscopic water testing has had a more significant impact on environmental sensing than all other photonic applications combined.1
During much of that time, in situ UV water sensors were limited in deployment to stationary continuous-flow monitoring stations. This is mainly due to size, power consumption, and the relatively short lifetime of traditional UV light sources. Fortunately, ultraviolet-C (UV-C) LEDs have matured and now tout typical lifetimes greater than 1000 hours of continuous operation. More importantly, they show little to no degradation with repeated on/off cycles—unlike traditional emission lamps—resulting in years of useful life under typical operating conditions. With most water monitoring systems running at very low duty cycles, this means a typical UV-C LED could easily last for 5 to 10 years under normal operating conditions.
In September 2020, Laser Focus World sister publication LEDs Magazine featured a detailed review of the benefits of UV-C LEDs for water testing.2 In the article, author Hari Venugopalan expertly laid out the rationale behind the shift from traditional broadband UV light sources, such as deuterium and xenon lamps, to UV-C LEDs for water analysis. He explained how LED technologies allow designers to “[simplify] the optical design, eliminating mirrors, photodiode arrays, and shutters, while enabling the use of inexpensive photodiodes.” He also discussed which LED wavelengths should be selected for various water contaminants, including nitrates and polyaromatic hydrocarbons (see table). However, the article is limited to intrinsic UV spectral properties of various water contaminates. In reality, this only scratches the surface of in situ UV water testing capabilities.In parallel to the advance in off-the-shelf UV-C LEDs, there have also been major developments in microfluidics and miniaturized chromatography, making portable “wet chemical sensors” for UV water analysis technologically viable. While the term may seem counterintuitive, especially when referring to water analysis (where all the samples are wet), in this context the terminology refers to the introduction of reagents to the water sample through microfluidic channels to form UV-active derivates. UV sensor capabilities are then expanded to quantify other common contaminants through these reactions, particularly nitrite levels.
Since different types of bacteria convert ammonia to nitrite and others convert nitrite to nitrate, it is important that a water sensor accurately quantitate the induvial concentrations of nitrites and nitrates, not just the total nitrogen content of water. EPA guidelines for maximum contaminate levels in water for nitrates are 10mg/L. In contrast, they are only 1 mg/L for nitrites, so a system that can only detect nitrates or total nitrogen may end up approving water as safe, but actually is contaminated with unacceptable nitrite levels.
As a result, there is ever-growing interest from both academic and commercial sectors in developing portable and handheld UV water testing instrumentation. To fully understand the next generation of portable UV water sensors, it is necessary to have a more holistic understanding of how UV-C LEDs and photodetectors are being applied in conjunction with recent microfluidics and chromatography technology developments.
Microfluidics in portable UV water sensors
The most accepted definition of a microfluidic device is one in which liquid flows through one or more channels under 1 mm in diameter. A rigorous, physical description of the exact dynamics within microfluidic channels is beyond the scope of this article. Still, it is important to understand that the narrower the channel, the more laminar the flow. While turbulent flow does have some advantages—for example, it is excellent for mixing—the resultant temporal-spatial heterogeneities within the sample volume often lead to measurement uncertainty. In contrast, microfluidic channels producing uniform laminar flows of uniform droplets have several advantages, including smaller sample requirements, decreased reagent consumption, lower limits of detection, lower optical power requirements, and air/oil gapped droplet formation.
The narrow path length and low overall volume of microfluidic devices have excellent optical properties, but require active pumping to generate flow. The most common type of pumping, known as electroösmotic pumping, uses an applied voltage across the channel to generate flow, taking advantage of the surface ions within the channel walls. Unfortunately, this methodology typically requires rather large voltages as well as more expensive materials, making it less than ideal for portable instrumentation. Alternatively, peristaltic and Venturi pressure pumps have reduced in size and cost over the years, to the point where they are now more than viable for integration into portable devices.
To demonstrate the effectiveness of these devices, a research team lead by James Grinias at Rowan University (Glassboro, NJ) recently showed that 3D-printed peristaltic pumps (costing around $80) and low-cost commercial Venturi pumps (costing around $100) could “provide stable flow (<2% RSD) over a range of 1 to 7 mL/min and high reproducibility in signal intensity at a droplet generation rate around 0.25 Hz (<3% RSD), which are comparable in performance to similar measurements on standard syringe pumps.”3 In the same article, Grinias and his team went on to verify that both low-cost pumps performed equivalently to more traditional syringe pumps used in laboratory microfluidics with a fluorescent microscope and a xenon lamp (see Fig. 1).
Using a similar homemade peristaltic pump, Xize Niu and his teams at the University of Southampton and SouthWestSensor Ltd. (both in Southampton, England) developed a field-deployable microfluidic UV water sensor that fits into a watertight cylinder 102 mm in diameter and 226 mm in length.4 Their device is based on a single-pump, three-channel design in which a water sample is pumped and combined with the reagent via a T-junction and introduced into a third channel containing oil for droplet formation. Two different UV LED absorption cells are incorporated since the reagent reacts with nitrite and nitrate in the water at different speeds. The first cell quickly measures absorption to detect the nitrite concentration. Then, a heater is used to accelerate the reaction with the nitrate before the second absorption cell measures the combined concentration (see Fig. 2).Their findings, published in Environmental Science & Technology, showed extremely high accuracy (6% error) during a three-week field deployment in a tidal river. The sensor shows the effects of tidal variations, rain, and other environmental effects on nitrate/nitrite concentration. According to the researchers, “This droplet microfluidic-based sensor is suitable for a wide range of applications, including monitoring of rivers, lakes, coastal waters, and industrial effluents.”
Chromatography UV water sensors
Chromatography is a broad term that encompasses adhesive-based chemical separations; a classic example is watching black ink separate into its constituent pigments when a paper gets wet. Most systems rely on specially designed columns for analytical applications, which causes various liquid or gas phase chemical species to separate out in time as they flow through. From a measurement standpoint, this adds an extra dimension from which absorbance or fluorescence data can be analyzed. However, from an instrumentation perspective, the overall photonic system requirements are virtually identical to the droplet microfluidics previously discussed. Therefore, it should not come as much of a surprise that the same developments in low-cost UV-C LED and pumps are also fueling the development of portable and handheld UV-enabled chromatography instrumentation. For example, collaborators from TE Laboratories Ltd. (Carlow, Ireland), Dublin City University, and the University of Tasmania (Hobart, Australia) developed and deployed a portable ion chromatography system using 3D-printed pumps and a UV-C LED detection system at 235 nm to detect nitrite and nitrate in septic, waste, and stream water.5 With this portable system weighing only 11 kg, they reported an impressive analytical range from 0.05 to 30 mg/L for nitrite and 0.10 to 75 mg/L of nitrate.
Perhaps even more exciting, a collaboration between teams at the University of Valencia (Valencia, Spain) and The National Autonomous University of Honduras (Tegucigalpa, Honduras) produced a “hand-portable” nano liquid chromatography (nanoLC) instrument utilizing a 255 nm LED for absorbance measurements.6 While the team did not report on the nitrite/nitrate detection levels to enable a direct comparison, they did demonstrate astoundingly low ng/mL level limits of detection for theobromine, theophylline, and caffeine levels in the water, thus showing great promise for truly handheld UV-enabled chromatography sensors to be used for environmental sensing.
As governmental and non-governmental organizations around the globe continue to push for higher and higher water quality standards around the world, there is no doubt UV-C LEDs will continue to play a critical role. In addition, it is a safe assumption that the next generation of UV-enabled microfluidic water testing equipment will contain multiple fluidic channels and multiple detection wavelengths. As a result, LEDs photodetectors, filter technology, and CMOS photodiode arrays will become as crucial to portable water testing as LCDs, backlights, and Bayer filters have to consumer electronics.
REFERENCES
1. K. S. Johnson and L. J. Coletti, Deep-Sea Res. (2002); doi.org/10.1016/s0967-0637(02)00020-1.
2. H. Venugopalan, “UV-C LEDs enable new frontiers in water quality monitoring,” LEDs Magazine, 126, 26–27, 31 (Sep. 2020); http://bit.ly/LEDsMagUVC.
3. J. J. Davis et al., Analytica Chimica Acta. (2021); doi.org/10.1016/j.aca.2021.338230.
4. A. M. Nightingale et al., Environ. Sci. Technol. (2019); doi.org/10.1021/acs.est.9b01032.
5. E. Murray et al., Talanta (2020); doi.org/10.1016/j.talanta.2020.120955.
6. H. D. Ponce-Rodríguez et al., Sci. Total Environ. (2020); doi.org/10.1016/j.scitotenv.2020.140966.
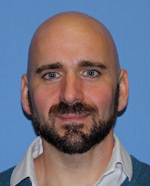
Robert V. Chimenti | Director, RVC Photonics LLC
Robert V. Chimenti is the Director of RVC Photonics LLC (Pitman, NJ), as well as a Visiting Assistant Professor in the Department of Physics and Astronomy at Rowan University (Glassboro, NJ). He has earned undergraduate degrees in physics, photonics, and business administration, as well as an M.S. in Electro-Optics from the University of Dayton. Over a nearly 20-year career in optics and photonics, he has primarily focused on the development of new laser and spectroscopy applications, with a heavy emphasis on vibrational spectroscopy. He is also very heavily involved in the Federation of Analytical Chemistry and Spectroscopy Societies (FACSS), where he has served for several years as the Workshops Chair for the annual SciX conference and will be taking over as General Chair for the 2021 SciX conference.