A tunable microlaser that emits two polarized circular beams directed at different angles?
How do artificial massive photons with spin, created within a specially designed optical cavity, emit light?
Curiosity about this question inspired scientists from the University of Warsaw in Poland, the Military University of Technology in Poland, and the University of Southampton in the UK to develop a new kind of birefringent optical microcavity filled with a liquid crystal.
“The microcavity consists of two perfect mirrors located so close to each other that a standing electromagnetic wave is formed inside,” says Jacek Szczytko, a physics professor at the University of Warsaw. “By applying electric voltage across the cavity, the liquid crystal molecules inside can be rotated.”
In previous works, the team demonstrated photons trapped within such an optical cavity behave like massive quasiparticles endowed with a magnetic moment called “spin.” In optics, the role of spin is played by the polarization of light.
Complex polarization patterns
These quasi-massive photons “can be exposed to an artificial magnetic field, they can form complex polarization patterns resembling exotic ferromagnetic phases known as half-skyrmions or merons (see Fig. 1), and recently we showed they behave like they are scattered by the Young’s double slits placed in reciprocal (Fournier) space,” he explains. “Generally, these photons are exotic and extravagant.”
They were curious what would happen if such a cavity emits light: do the photons inherit their massive and spin-like character? To find out, they filled their liquid-crystal microcavity with 1–2% laser dye.
As far as the basic optics involved, “the light within the cavity interacts with the molecules in a different way when the electric field of the propagating wave oscillates along the molecules and also when oscillations are perpendicular to them,” says Wiktor Piecek, head of the Laboratory of Crystal Physics and Technology at the Military University of Technology’s Institute of Applied Physics.
The liquid crystal is a birefringent medium—it can be characterized by two refractive indexes, which depend on the direction of the electric field oscillations or electromagnetic wave polarization.
Two polarized circular beams directed in different angles
When the team precisely arranged molecules inside the laser microcavity, it resulted in the appearance of two linearly polarized light modes within the cavity—two standing waves of light with opposite linear polarizations (see Fig. 2).The electric field applied to the electrodes deposited on the microcavity mirrors changed the orientations of the molecules inside the optical cavity. “Consequently, this changed the effective refractive index of the liquid crystal layer and controlled the length of the optical path of light—the product of the width of the cavity and the refractive index on which the energy (color) of the emitted light depends,” says Piecek. “One of the modes didn’t change its energy as the molecules rotated, while the energy of the other increased as the orientation of the molecules changed.”
By optically stimulating the organic dye placed between the molecules of the liquid crystal, the team obtained a lasing effect—coherent light radiation with a strictly defined energy. “The gradual rotation of the liquid crystal molecules led to unexpected properties of this lasing,” says Marcin Muszyński, a PhD student at the University of Warsaw.
Lasing was achieved for this tunable mode: the laser emitted one linearly polarized beam perpendicular to the surface of the mirrors. “Using liquid crystals enabled smooth tuning of the light wavelength with the electric field by as much as 40 nm,” Muszyński adds.
Perhaps the most surprising aspect of their work came when the team rotated the liquid crystal molecules so both energy modes—the one sensitive to the orientation of the molecules and the one that didn’t change its energy—were in resonance. “Light emitted from the cavity suddenly changed its polarization from linear to two circular beams: right- and left-handed, with both circular polarities propagating in different directions, at an angle of several degrees,” explains Szczytko.
And phase coherence of the laser was confirmed in an interesting way. “A persistent-spin helix—a pattern of stripes with different polarized light, spaced about 3 µm apart—appeared on the surface of the sample,” says Muszyński.
Theoretical calculations show such a pattern can be formed when two oppositely polarized beams are phase coherent and both modes of light are inseparable—and this phenomenon can be compared to quantum entanglement.
The biggest challenge is “the laser works in pulses, so far, because the organic dye we used slowly photodegrades under the influence of intensive light,” Muszyński points out. “We hope replacing the organic emitter with more durable polymers or inorganic materials, such as perovskites, will allow for a longer lifetime.”
This precisely tunable laser—without discrete optical modes known from other types of tunable lasers—can be used within many fields of physics, chemistry, medicine, and communications.
“We use nonlinear phenomena to create a fully optical neuromorphic network,” says Barbara Pietka, a physics professor at the University of Warsaw. “This new photonic architecture can provide a powerful machine-learning tool for solving complex classification and interference problems, and for processing large amounts of information with increasing speed and energy efficiency.”
Next up, the group will use unique properties of their birefringent microcavity to enforce the emission from topologically protected states of light. “These states are different than normal ‘trivial’ states,” says Szczytko. “For instance, they form unidirectional flow robust against backscattering and vortex states carrying quantized angular momentum.”
They plan to tap into the strong nonlinearities observed in organic microcavities and optical spin-orbit coupling in liquid-crystal cavities to demonstrate single-photon polarization switches for binary and tertiary logic. “Our organic liquid-crystal microcavities platform will combine a strong emissivity with the ease of fabrication, low costs, scalability, and room-temperature operation,” Szczytko adds.
This work received funding from the European Union FET-Open H2020 Project TopoLight and the National Science Centre of Poland. The team is now seeking opportunities to commercialize their technology.
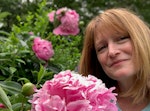
Sally Cole Johnson | Editor in Chief
Sally Cole Johnson, Laser Focus World’s editor in chief, is a science and technology journalist who specializes in physics and semiconductors.