A recent collaboration led by University of Innsbruck researchers in Austria revealed that hot (alive) Schrödinger cat states can indeed be created within a superconducting microwave resonator—and it means quantum phenomena can be generated and used within less perfect and warmer conditions than previously believed.
Schrödinger cat states are arguably one of the most intriguing phenomena within quantum physics and it’s why a “cat within a box” is commonly used as a memorable way for physics professors to show that a quantum object can exist simultaneously within two different states. You don’t know the cat’s state—alive or dead until you open the box—so it’s essentially both alive and dead simultaneously.
This type of simultaneity can be seen during real experiments within the locations of atoms and molecules and the oscillations of electromagnetic resonators. Before now, to achieve these states a quantum object had to be cooled to its ground state (the lowest energy state within the system).
Schrödinger’s original thought experiment assumes hot cat states, and the researchers wanted to explore whether these quantum effects can be generated without starting from a cold ground state. Their breakthrough demonstrates quantum superpositions can be created from thermally excited states.
“Our experiment is one of these ‘happy accidents’ that only happen within a collaborative environment where scientists talk with each other frequently,” says Professor Gerhard Kirchmair of the Institute for Experimental Physics, as well as senior group leader and managing director of the Austrian Academy of Sciences’ Institute for Quantum Optics and Quantum Information. “This all started when my colleague Oriol Romero-Isart (director of the Institute for Photonic Sciences in Spain) told me over coffee they’d seen theoretically that cat states can be generated out of a thermal state.”
Kirchmair had wondered about this possibility but hadn’t pursued it. But when Romero-Isart and his student Thomas Agrenius discussed their ideas conversation with Kirchmair within the context of mechanical systems being difficult to cool, they realized quantum superpositions can be created within such a “hot” system—and these discussions kicked off the group’s experiments.
How are ‘hot’ Schrödinger cat states created?
The group’s design involves a high coherence microwave cavity coupled to a superconducting qubit—which forms a circuit quantum electrodynamics system. The interaction between the two systems enables running conditional operations on each of the systems. In other words: It conditionally displaces the cavity depending on the state of the qubit or flips the qubit’s state depending on a certain number of photons within the cavity. One condition required for this to work is that both the qubit and cavity have large enough coherence times.
To achieve it, Kirchmair and colleagues exploit running these conditional operations between the cavity and qubit to create a cat state. “The hot cat state is created by first ‘heating up’ the cavity by injection noise created by a hot resistor at room temperature,” he explains. “We then run the same conditional operations on the system slightly modified to account for the larger photon numbers due to the noise.”
They use two special protocols to create their hot Schrödinger cat states, which they’d previously used to produce cat states starting from the ground state of the system. These adapted protocols also “work at higher temperatures and generate distinct quantum interferences,” says Romero-Isart, which opens the door to “the creation and use of quantum superpositions within nanomechanical oscillators, for which achieving the ground state can be technically challenging.”
Ian Yang, a Ph.D. student at the time and now a postdoctoral researcher at Paul Scherrer Institute in Switzerland, ran the group’s experiments and achieved quantum superpositions at temperatures reaching 1.8 Kelvin—60x hotter than the cavity’s ambient temperature. This shows “it’s possible to generate highly mixed quantum states with distinct quantum properties,” he says.
While heat typically destroys quantum effects, Agrenius points out that the group’s measurements confirm quantum interference can persist even at high temperatures.
And this is the key takeaway of their work: Quantum superposition can be generated regardless of the temperature of the systems—as long as it’s like a harmonic oscillator or most mechanical systems and resonators—with the right interactions available and a cold quantum system as a resource.
These findings should help enable the development of quantum technologies. “Our work reveals it’s possible to observe and use quantum phenomena even within less ideal, warmer environments,” says Kirchmair. “And if we can create the necessary interactions within a system, temperature ultimately doesn’t matter.”
One challenge that had to be overcome was to “understand that the sequence needs to change to account for the larger number of photons within the system due to the thermal excitation,” says Kirchmair.
The coolest aspect of this work for Kirchmair? Figuring out there’s more than one type of hot cat state. “We’ve shown there are two possibilities for the superposition, which don’t show up in the cold case,” he says. “Originally, our theory colleagues had one type of hot cat in mind but we initially always created the other one. During the course of our experiment we realized different symmetries are possible between the two states of superposition, and it helped us to understand that different states exist.”
Quantum sensing can benefit
Quantum sensing can benefit from this work because it shows cat states can be created—which are good for quantum-enhanced sensing—even without ground-state cooling. “A mechanical oscillator system (a good acceleration or force sensor), for example, is normally cooled to the ground state to achieve large sensitivity,” says Kirchmair.
These cat states are also used for quantum error correction approaches, which means they must be reliably created. “We show that residual thermal population doesn’t pose a limitation for creating these states,” Kirchmair says.
What’s next for the group? “We’ll use the system for what it was originally designed—to mediate interactions between multiple qubits for novel quantum gates,” says Kirchmair.
FURTHER READING
I. Yang et al., Sci. Adv., 11, eadr4492 (2025); https://doi.org/10.1126/sciadv.adr4492.
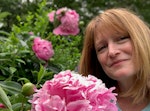
Sally Cole Johnson | Editor in Chief
Sally Cole Johnson, Laser Focus World’s editor in chief, is a science and technology journalist who specializes in physics and semiconductors.