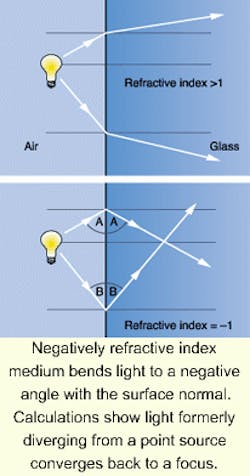
Conventional wave optics dictates that light going through an optical lens can never create an image quite as good as the original, because light cannot be focused to a point smaller than the square of its wavelength. The image viewed is essentially a grainy and washed-out version of the source. But physicist John Pendry of Imperial College (London, England) recently challenged this traditional limitation by showing that a negative refractive index may enable a superlens capable of focusing light to a point hundreds of times smaller than its wavelength. Practical applications of such a lens could be found in medical imaging (MRI) or lithography.
A change in refractive index is what causes light to bend when passing from one material into another. The typical value of the refractive index in glass, n = 1.4, causes light to converge to a focus. But even the best conventional lenses are unable to focus all the light rays, due to the decay of evanescent waves within a few nanometers of the source. A refractive index of less than one indicates that the light bends in the opposite direction, towards the boundary. This is known to occur for x-rays, but is uncommon for longer-wave radiation such as light and radio. Pendry's proposal is even more radical—a refractive index of less than zero (see figure).
Pendry used Maxwell's equations of electromagnetic waves to calculate that evanescent waves are not lost when passing through a hypothetical material with a refractive index of n = -1. The calculations show two solutions for a material with a negative refractive index, the second of which allows near-field evanescent waves to grow instead of decaying. This effect can exactly balance out the decay, ensuring that the information is perfectly restored in an image of the source. In such a material, the angle of deflection of the light ray would be equal to the angle of incidence, but in the opposite direction. A flat slab of such a material would send all the rays originating from a single point back to a mirror-image point on the other side of the boundary. It would be a perfect lens without the demand for curved surfaces.
The problem with the idea is that there are no known materials with such a negative refractive index for visible light. A device made of thin gold wires designed earlier this year by Sheldon Schultz at the University of California, (San Diego, CA) demonstrated a negative refractive index within the microwave region. And in collaboration with Hammersmith Hospital in London, Pendry has designed a negative-refraction index lens for radio waves for focusing in magnetic-resonance imaging.
"More conventional materials might make perfect lenses if the electromagnetic properties were tuned just right," said Pendry. "A very thin film of silver, 40 nm thick, behaves like a nearly perfect plasma with the proper dielectric properties and just might work." Pendry and colleagues have begun initial investigation into these flat, thin-film, silver lenses with promising results.
A major obstacle to widespread practical use of such negatively refractive lenses is that they require placement only nanometers from the source and would refocus light within a very short distance. This limits its use to small-scale applications, such as improving the precision with which a compact disc can be written and read, thus increasing the amount of information that can be stored, currently limited by the wavelength of light.
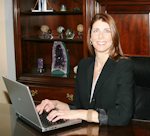
Valerie Coffey-Rosich | Contributing Editor
Valerie Coffey-Rosich is a freelance science and technology writer and editor and a contributing editor for Laser Focus World; she previously served as an Associate Technical Editor (2000-2003) and a Senior Technical Editor (2007-2008) for Laser Focus World.
Valerie holds a BS in physics from the University of Nevada, Reno, and an MA in astronomy from Boston University. She specializes in editing and writing about optics, photonics, astronomy, and physics in academic, reference, and business-to-business publications. In addition to Laser Focus World, her work has appeared online and in print for clients such as the American Institute of Physics, American Heritage Dictionary, BioPhotonics, Encyclopedia Britannica, EuroPhotonics, the Optical Society of America, Photonics Focus, Photonics Spectra, Sky & Telescope, and many others. She is based in Palm Springs, California.