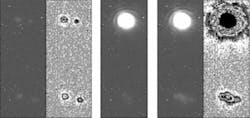
Large extrasolar planets can be detected by the dip in intensity they cause as they pass in front of the star they orbit, or by the wobble they induce in the star’s position. But as much as we like to find gas giants orbiting other stars, we would more like to find an Earth-size planet in a sensibly sized orbit—and to spectroscopically analyze its atmosphere for signs of life. To do this, the light reflected from the planet must be made visible against the star’s prodigious output.
The best approach to date is to interferometrically null the star’s output while letting the planet’s light shine through. While interferometers are a natural for monochromatic light, the light from a star and its planet is broadband—thus spurring the development of achromatic interferometer-coronagraphs based on the non-common-path Michelson interferometer. However, these non-common-path interferometers are sensitive to the slightest mechanical instabilities.
Geometrical phase shift
Researchers at the Tokyo University of Agriculture and Technology and the University of Electro-Communications (both of Tokyo, Japan) have developed an achromatic interferometer-coronagraph for extrasolar-planet detection that has a common path to allow stable operation under nonideal conditions.1 The 180° rotational-shearing interferometer, which has a 3-D path and is a modified Sagnac interferometer, introduces a geometrical π phase shift for an on-axis source, nulling the source. Off-axis sources have reduced interference, allowing the sources to be seen.
In the optical train, a broadband beamsplitter polarizes the incoming on- and off-axis source light, which then passes through a second broadband polarizing beamsplitter with its polarization axes out of plane; the two resulting beams are directed into and though a common mirror system in opposite directions. The mirror system geometrically rotates the images by 180° with respect to each other, and thus also the electrical vectors, producing the π on-axis phase shift.
Light from off-axis sources actually produces two images, which don’t interfere (if the images overlap at all, then some of the light does destructively interfere, but the rest does not). Non-nulled (off-axis) light is diverted by the second beamsplitter to an imager.
For the on-axis source in their experiment, the researchers used a pinhole-filtered halogen lamp that resulted in a source with an angular size of 4 arc min, a spectral width of 300 nm, and a center wavelength of 500 nm. The off-axis source was a single-mode-fiber-coupled superluminescent diode with a 20-nm spectral width and a smaller angular size. A CCD camera captured the result (see figure). To demonstrate the mechanical stability of the system, the CCD camera was set to integrate for four minutes.
Real stars and extrasolar planets would have far smaller angular sizes than the experimental sources. The smaller the angular size, the better the nulling; while the experimental sources could be nulled to 10-3, a real star could, with the same setup, be nulled to 10-10. The group is now working with white-light sources 0.2 arc min in angular size, says Alexander Tavrov, one of the researchers.
The experimental results agreed well with computer simulations, and showed that the residual nonnulling of the on-axis source was due to the extended on-axis source size and not to insufficient achromaticity of the interferometer. Other potential applications include microscopy, says Tavrov.
REFERENCE
1. A. V. Tavrov et al., Optics Lett.30(17) (Sept. 1, 2005).
About the Author
John Wallace
Senior Technical Editor (1998-2022)
John Wallace was with Laser Focus World for nearly 25 years, retiring in late June 2022. He obtained a bachelor's degree in mechanical engineering and physics at Rutgers University and a master's in optical engineering at the University of Rochester. Before becoming an editor, John worked as an engineer at RCA, Exxon, Eastman Kodak, and GCA Corporation.