Photonic Frontiers: beam combining - Combining beams can boost total power
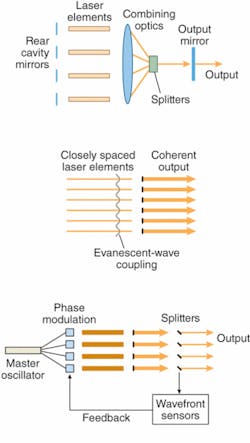
Efforts to scale the output power of a single-output laser inevitably run into limits. As laser size increases, so does the difficulty of removing waste heat, maintaining beam quality, and avoiding optical damage to output optics. Those limits are particularly stringent for solid-state and semiconductor lasers—but there is an alternative. Combining beams from many small laser elements can produce a single higher-power beam.
Diode-laser arrays have long generated high powers by combining the outputs of many laser stripes. That works well for applications, like diode pumping, that do not require high beam quality. However, combining outputs in that way cannot increase the radiance beyond that of a single laser stripe. “The ‘holy grail’ of beam combining is to take a bunch of lasers and get a single Gaussian beam out,” says T. Y. Fan of the MIT Lincoln Laboratory (Lexington, MA). A large military research program 15 to 20 years ago tried to combine diode-laser beams, but met with little success. Now researchers have returned to the task of beam combination with a much better understanding of the process, and despite formidable challenges they are making considerable progress.
Methods of combining beams
In an excellent tutorial review, Fan divides beam combiners into three broad classes.1 The most simple and established types are side-by-side combiners such as diode arrays, which make no effort to control the phase or wavelength of the separate emitters. Side-by-side combiners suffice for applications requiring raw power, such as diode pumping, but their overall beam quality is poor and the intensity can’t exceed that of the individual sources.
The primary approach to better beam combination has long been the optical counterpart of phased-array radar, which adjusts the phases of beams from separate laser elements to enable them to combine coherently. The goal is to make the electric-field amplitudes add constructively to generate a single high-quality Gaussian beam. However, it has proved difficult to adjust phases accurately enough to generate a high-quality beam, particularly when combining many separate beams. Complications include the need to control polarization, amplitude, and power spectra of the individual elements so they combine properly.
A second approach that has gained popularity recently is to combine beams of different wavelengths to generate high power across a broad spectral range. The obvious inspiration is wavelength-division multiplexing in optical fibers. Instead of shifting the phase of light from separate emitters, the beam-combining optics superimpose the separate wavelengths, allowing their powers—instead of their wave amplitudes—to add. This incoherent approach relaxes alignment tolerances and promises better combination of beams from more elements, but the combined beams span a range of wavelengths, which may not be desirable in some applications.
Coherent beam combining
Controlling the phase of the light waves emitted by the separate elements so their amplitudes add properly is the biggest problem with coherent beam combination. A second key issue is how much of the collecting field is filled by light-emitting elements; the lower the fill factor, the more difficult combination becomes.Coherent combination has been demonstrated with diode, solid-state, fiber, and gas lasers, but current efforts focus on diode and fiber lasers. A number of combination arrangements are possible (see Fig. 1). In one arrangement, several laser modules with separate rear-cavity mirrors can be optically coupled to a single output-coupling mirror, so the common resonator generates a single beam, but precisely matching path lengths of the elements is difficult. Another alternative is to space many separate laser elements closely enough for their oscillating fields to overlap, which creates evanescent-wave coupling, but in practice adjacent elements tend to couple 180° out of phase and scaling to large sizes is difficult. Other configurations use nonlinear optics or depend on active feedback to control the phase of each element.
A big benefit of coherent coupling is high spectral brightness, because output is at the same wavelength. However, maintaining that coherence is tricky. Path lengths can change many waves as the laser heats to operating temperature, and after the laser reaches steady-state operation path lengths can vary by an appreciable fraction of a wavelength in a quiet and well-controlled room.
Another issue is that coherent combination of arrays with low fill factor—inevitable in arrays with many elements—tends to concentrate much of the power in side lobes. At CLEO (San Jose, CA) last May, Mercedeh Khajavikhan and James Leger of the University of Minnesota (Minneapolis, MN) showed that an afocal imaging system with a pair of customized phase plates could reduce side lobes and concentrate power in the main lobe (see Fig. 2).2
Other developers also reported encouraging results at CLEO. Robin Huang of Lincoln Laboratory reported combining outputs of ten independently addressable diode-laser stripes in a 980 nm slab-coupled optical-waveguide laser (SCOWL) array to generate a single beam with continuous-wave power of 7.2 W. His group used an external Talbot cavity, an array of diffractive optical elements that adjusts input beam phases and combines them. Changing drive currents of elements by 2 to 5 mA was enough to lock them in phase.3
Stimulated Brillouin scattering in optical fibers also can clean up beams for coherent combination because it forms gratings aligned with all fiber modes. At CLEO, Laurent Lombard and colleagues from ONERA, the French national aerospace laboratory (Palaiseau, France) reported that stimulated Brillouin scattering in graded-index fiber cavities coherently combined beams from two fiber amplifiers.4
Wavelength/spectral combining
Wavelength or spectral beam combination incoherently adds the powers of the input beams and sums their spectral ranges. So far, this method has generated higher total power than coherent combination, although only with a few bright input beams. Spectral combination produces a high-quality Gaussian beam in near and far fields, without strong side lobes, making it attractive for applications requiring high total power rather than peak intensity at a specific wavelength.Combining beams from ytterbium-doped fiber lasers emitting at different wavelengths has yielded several hundred watts in a single beam. Last year, Thomas H. Lofton and colleagues at Aculight (Bothell, WA) described two experiments using diffraction gratings oriented for Littrow reflection. The collimated 3.6 mm input beams were aligned at angles chosen so the grating reflected them all in the same direction to form a single beam (see Fig. 3). Combining two beams generated an average power of 323 W with 93% efficiency and beam-quality factor M2 of 1.16. Similar experiments with three beams generated average power of 522 W with 93% efficiency and M2 of 1.18.5
Early this year, Leonid Glebov’s group at the University of Central Florida (Orlando, FL) set a new power record by combining five continuous-wave ytterbium-fiber laser beams to produce more than 750 W in a single near-diffraction-limited beam.6 The group used highly dispersive volume Bragg gratings in photorefractive glass, which strongly reflect a narrow range of wavelengths and transmit surrounding bands. The researchers combined beams by adding new wavelengths sequentially (see Fig. 4). Each Bragg filter was tuned to transmit wavelengths already in the beam and reflect the wavelength being added. Efficiency reached 93% in combining wavelengths from 1062.08 to 1064.55 nm, limited by crosstalk and material losses. Modeling results of combining a couple of hundred input beams, the group found that achieving 90% efficiency would require limiting material loss to 0.0006 per centimeter and crosstalk to 1%.Outlook
Although recent results are encouraging, big challenges remain. So far the highest powers have come from combining only a few powerful fiber sources. Combination of larger arrays of laser elements to produce good quality beams has been demonstrated only at lower powers. Other challenges include balancing the tradeoffs between diode arrays and arrays of fiber lasers, reducing the light lost to side lobes, and efficiently combining light from input arrays with relatively low fill factors.
The big questions are how high can the power go, and how well will it scale? Will beam combining work best for a few comparatively powerful fiber elements, or will the total power and beam quality be higher if many lower-power beams are combined? How well will beam combination compete with large single laser elements? In one high-profile shoot-out—for development of solid-state tactical laser weapons—single-aperture lasers currently hold the lead for raw power and are expected to reach 100 kW first. But beam combination from high-power fiber lasers is coming on strong. Tomorrow’s bullets of light might come from arrays of fiber lasers.
REFERENCES
1. T.Y. Fan, IEEE J. Selected Topics in Quant. Electronics 11, 567 (May/Jun 2005).
2. M. Khajavikhan and J.R. Leger, CLEO 2008, paper CWB1.
3. R.K. Huang et al., CLEO 2008, paper CMN1.
4. L. Lombard et al., CLEO 2008, paper CTuB3.
5. T.H. Lofton et al., IEEE J. Selected Topics in Quant. Electronics 13, 487 (May/Jun 2007).
6. A. Sevian et al., Optics Letters 33, 384 (Feb 15, 2008).
Editor’s note: For more on beam combining, see “High-power fiber-laser beams are combined incoherently” in August on our Web site at www.laserfocusworld.com/articles/331428.
About the Author
Jeff Hecht
Contributing Editor
Jeff Hecht is a regular contributing editor to Laser Focus World and has been covering the laser industry for 35 years. A prolific book author, Jeff's published works include “Understanding Fiber Optics,” “Understanding Lasers,” “The Laser Guidebook,” and “Beam Weapons: The Next Arms Race.” He also has written books on the histories of lasers and fiber optics, including “City of Light: The Story of Fiber Optics,” and “Beam: The Race to Make the Laser.” Find out more at jeffhecht.com.