PHOTONIC FRONTIERS: WAVELENGTH CONVERSION - All-optical: the path to high-speed wavelength conversion
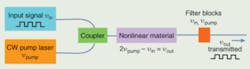
To change the wavelength of a signal, today’s wavelength-division-multiplexed networks have to convert optical signals to electronic form to drive a laser transmitter emitting at the desired wavelength. The dramatic success of optical amplifiers makes that optoelectronic approach seem cumbersome, and for more than a decade developers have been seeking all-optical wavelength converters for optical networks.
The two leading approaches have evolved from ideas that emerged a decade ago. Wavelength mixing in a nonlinear medium promises low noise, very high speed, and low loss, but requires high nonlinearity and is relatively bulky. Converters based on semiconductor optical amplifiers (SOAs) are more compact and easier to integrate, but suffer from higher noise and need careful design to achieve high speed. The two can be combined by mixing waves inside an SOA.
The good news is that some impressive results have been achieved with various approaches. But all-optical wavelength converters are not ready for deployment because the desirable features have yet to be combined into a practical device. And the requirements for future optical networks are still evolving. Future networks will require integration of wavelength conversion with optical regeneration and switching, and high-speed operation may require optical-packet switching and optical-burst switching.
The need for speed
The need for higher network capacity is driving development. Optical-electronic-optical (OEO) wavelength conversion suffices at 10 Gbit/s. Intense development has made integrated photonics cheap, at least relative to the complexities of all-optical wavelength conversion. Although OEO wavelength converters require a receiver and laser transmitter, the electronics are straightforward to build at 10 Gbit/s.
Both electronics and optics are contenders for 40 Gbit/s networks, but the balance shifts at higher speeds. “When you get to 100 gigabits and above, you get to the realm where electronics would be very difficult,” says Alan Willner of the University of Southern California (Los Angeles, CA). All-optical wavelength conversion promises much faster speeds. The cutting edge demonstrated in the laboratory has reached an impressive 320 Gbit/s.
Basic concepts
The major approaches for all-optical wavelength conversion date back a decade, but have been considerably refined, says S. J. Ben Yoo of the University of California at Davis (Davis, CA). The four main variations are parametric conversion in a highly nonlinear material, parametric mixing in a semiconductor optical amplifier (SOA), cross-phase modulation in an SOA, and cross-gain modulation in an SOA.
Parametric conversion combines photons from a strong pump beam with the input signal beam to generate a third wavelength. In four-wave mixing, two pump-beam photons combine with an input photon to generate an output photon:Alternatively, three-wave mixing produces a new wavelength by difference-frequency generation, and filters can block the undesired wavelengths (see Fig. 1).
Efficient parametric conversion in a nonlinear material requires the high nonlinearity of materials such as lithium niobate and highly nonlinear optical fibers. Noise is low because nonlinear materials do not produce spontaneous-emission noise, and photonic-crystal fibers can be made to have very high nonlinearity. However, nonlinear devices are made from discrete optics, making them hard to integrate-a significant disadvantage in system applications.
Alternative approaches are based on controlling SOAs to transfer modulation of the input signal to a different wavelength amplified by the SOA. Recovery times make amplifiers inherently slower than nonlinear devices, but careful compensation can achieve extremely high speed. In addition, SOAs can be integrated with other devices, and have internal amplification, important advantages for combining optical regeneration with wavelength conversion.
Parametric generation of a new wavelength from a pump beam and an input signal can be performed within an SOA, which then amplifies the generated wavelength to produce a wavelength-converted signal. Filters can remove undesired wavelengths.
Alternatively, the input signal can modulate amplification of a continuous-wave (CW) signal at the output wavelength. Cross-gain modulation depends on saturating an SOA’s gain at the output wavelength, provided by a continuous pump laser. The saturation when the input signal is high reduces the amplified power at the output wavelength by an amount proportional to the instantaneous input signal. The overall effect is to modulate the continuous output beam with the inverse phase of the input signal, generating zeros instead of ones and vice versa. The converted signal then is inverted to regain the original data stream.
Cross-phase modulation uses the input signal to modulate the phase of the CW pump beam rather than its amplitude in the SOA. In this case, the input beam depletes carrier density, which changes the refractive index of the semiconductor, and thus shifts the phase of the amplified light by an amount proportional to the instantaneous intensity of the input signal. Putting the output beam through an interferometer converts the phase modulation into intensity modulation. Early tests used a pair of SOAs and a Mach-Zehnder interferometer, but Juerg Leuthold of the University of Karlsruhe (Karlsruhe, Germany) says that following a single SOA with a delay interferometer can overcome the long recovery time that follows the SOA’s fast initial response. The delay interferometer splits the signal between a linear waveguide and a loop which delays the pulse by an interval of about 10 ps. Recombining the light from the two arms of the interferometer cancels the recovery tail to give a much shorter pulse (see Fig. 2).Semiconductor optical amplifiers have other advantages including low power consumption and operation across tens of nanometers in wavelength. Engineering challenges include polarization sensitivity and spontaneous emission noise.
320 Gbit/s demonstrations
Four recent demonstrations have shown wavelength conversion is possible at speeds up to 320 Gbit/s. Yong Liu and colleagues of the Eindhoven Institute of Technology (Eindhoven, The Netherlands) reached 320 Gbit/s using a single SOA followed by a fiber Bragg grating and two cascaded optical bandpass filters. The input data stream had an average power of roughly 4 mW, double the power of the CW input from a tunable laser. The input signal affects the CW laser input both by cross-gain modulation and by modulating the refractive index of the semiconductor, which adds a wavelength chirp to the output signal. To extract the output signal, they passed it through filters that selected a band blue-shifted from the CW laser input, which helped suppress slow gain recovery to give 1.8-ps gain recovery. Then they passed the inverted output through a delay interferometer to recover an uninverted signal.1
At CLEO 07 (Baltimore, MD; May 7-11), Bernd Huettl and colleagues at the Heinrich Hertz Institute (Berlin, Germany) demonstrated 320 Gbit/s wavelength conversion by combining an input signal at 1546.5 nm and a pump-laser beam at 1540.5 nm in a 93 mm waveguide of periodically poled lithium niobate. They cascaded two processes; frequency doubling of the pump beam and difference-frequency generation of the second harmonic and the input signal, producing an output signal at 1534.5 nm. The group reported converting both differential quaternary phase-shift-keying pulses at 320 Gbit/s and differential phase-shift-keying pulses at 160 Gbit/s.2
Also at CLEO 07, Michael Galili and colleagues of the Technical University of Denmark (Lyngby, Denmark) described 320 Gbit/s wavelength conversion using cross-phase modulation in highly nonlinear optical fiber. The group combined a CW beam at 1544 nm with a modulated beam at 1557 nm at one end of the fiber. A counterpropagating Raman pump enhanced conversion by generating Raman gain (see Fig. 3). Galili reported only 0.2 dB of power penalty using a 200 m length of fiber.3At OFC 07 (Anaheim, CA; March 26-30) Huettl and Galili reported a hybrid experiment, with four-wave mixing producing wavelength conversion of a 320 Gbit/s DQPSK (differential quadrature phase-shift keying) signal in a 1100 m length of highly nonlinear fiber.4
Competition with optoelectronic devices, outlook
A large gap separates the high-speed experiments from more practical devices. Bell Labs (Murray Hill, NJ) last year reported a wavelength converter monolithically integrated with a laser that can be switched among many wavelengths to generate specific output on demand, but its data rate was 40 Gbit/s.5 Integration and tunability are both essential for practical applications in future optical networks, but a host of tradeoffs remain to be weighed to extend them to higher speeds.
Other challenges also remain in adapting the devices to fit the applications. Wavelength processing will be part of the switching fabric needed for future high-capacity networks, says Yoo. He believes wave-mixing systems have an advantage in future networks because they offer greater transparency that may be essential for advanced switching.6 Network architecture may play a dominant role in selecting the winning technology.
But Yoo also notes that devices developed for wavelength conversion are not limited to that application. Broadly speaking, he says, wavelength converters are signal processors with an input, an output, and a control signal, like transistors or triodes from the vacuum-tube era. Those capabilities may find many more applications.
REFERENCES
1. Y. Liu et al., J. Lightwave Technology 24, 103 (2007).
2. B. Huettl et al., CLEO 07, paper CThF1.
3. M. Galili et al., CLEO 2007, paper CThF4.
4. M. Galili et al., OFC 2007, paper OTuI3.
5. Pi. Bernasconi et al., J. Lightwave Technology 24, 71 (January 2006).
6. S.J. Ben Yoo, J. Lightwave Technology 24, 4468 (December 2006).
Tell us what you think about this article. Send an e-mail to [email protected].
About the Author
Jeff Hecht
Contributing Editor
Jeff Hecht is a regular contributing editor to Laser Focus World and has been covering the laser industry for 35 years. A prolific book author, Jeff's published works include “Understanding Fiber Optics,” “Understanding Lasers,” “The Laser Guidebook,” and “Beam Weapons: The Next Arms Race.” He also has written books on the histories of lasers and fiber optics, including “City of Light: The Story of Fiber Optics,” and “Beam: The Race to Make the Laser.” Find out more at jeffhecht.com.