Researchers at the University of St. Andrews (Fife, Scotland) and Macquarie University (New South Wales, Australia) have demonstrated a compact, fluidic-fiber dye laser that operates without the need for external mirrors or lithographically defined microstructures.1 Lasing is based on capillary action within the tubes of a photonic-crystal (PC) fiber; the lasing wavelength can be tuned by varying the chromophore density in the liquid core.
The fluidic-fiber laser was fabricated by butt-coupling a pump capillary fiber to one of two different fibers (see figure). The first fiber was a single-core silica capillary tube from Polymicro Technologies (Phoenix, AZ) with a 2 µm inner core diameter and 128 µm cladding diameter. The second fiber was a PC fiber from Crystal Fibre (Birkerød, Denmark) that contained a hexagonal array of capillaries with hole diameters of 3.68 µm, a period of 8 µm, and a solid silica core with diameter 12 µm and overall cladding diameter 125 µm. The fibers were cleaved at appropriate lengths and dipped in the gain medium-a toluene solution of ionized perylene red organic dye at a concentration of 0.5 mg/ml. The infilling process due to capillary action was measured to be 1 µm/s for a 25-mm-long test capillary with a 5 µm hole diameter. The organic solvent was chosen to have a refractive index higher than silica.
To delay evaporation of the solvent, each of the fibers was capped by dipping them in a viscous polymer solution. A neodymium-doped yttrium vanadate (Nd:YVO4) laser with a 200 Hz repetition rate and a 7.5 ns pulse duration was first coupled to an 8-µm-core silica fiber that was then butt-coupled to either a 41-mm-long 2-µm-core fluidic capillary or to a 39-mm-long PC fluidic fiber.
Axial emission from the fibers was focused (with a 25× microscope objective) onto a fiber-coupled CCD spectrometer with 40 pm resolution. For both the 2-µm-core fluidic capillary laser and the multiple-core PC fluidic laser, the emission spectra at and above threshold are characterized by a series of narrow spectral peaks around 620 nm. However, the threshold energy and slope efficiency were different for single and multiple-core fluidic lasers of similar lengths, most likely due to the efficiency of coupling the pump light into the different fluidic lasers and also because the PC fiber has a larger active area, with multiple cores excited simultaneously. In addition, threshold and efficiency were dependent on fiber length. Because the emitted photons experience gain in a short section of fiber where the excitation is first absorbed (as short as 800 µm), the longer fiber lengths cause loss due to reabsorption of photons.
Vernier resonance
To understand why the measured free spectral range of these single and multicore capillary lasers is approximately 300 times larger than expected for a Fabry-Perot laser formed by the liquid-waveguide and dye-cavity interfaces, the researchers studied the dependence of the laser mode spacing as a function of the liquid length of the lasers. They found that a Vernier resonant effect caused by the presence of two or more cavities with different FSR values creates a spectral overlap that leads to a longer overall mode spacing.
“These lasers show an intriguing effect that by making the waveguides support a few transverse modes, we observe a dramatic reduction in the number of spectral modes that lase,” says researcher Graham Turnbull of the University of St. Andrews. “The underlying mechanism should provide a simple new way to achieve single-frequency operation in fiber lasers. By simply swapping the laser dye, the emission spectrum can be tuned across the visible, making these lasers convenient miniature sources for spectroscopy and chemical and biosensing.”
REFERENCE
1. A.E. Vasdekis et al., Optics Express 15 (7) 3962 (April 2, 2007).
About the Author
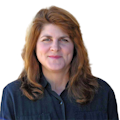
Gail Overton
Senior Editor (2004-2020)
Gail has more than 30 years of engineering, marketing, product management, and editorial experience in the photonics and optical communications industry. Before joining the staff at Laser Focus World in 2004, she held many product management and product marketing roles in the fiber-optics industry, most notably at Hughes (El Segundo, CA), GTE Labs (Waltham, MA), Corning (Corning, NY), Photon Kinetics (Beaverton, OR), and Newport Corporation (Irvine, CA). During her marketing career, Gail published articles in WDM Solutions and Sensors magazine and traveled internationally to conduct product and sales training. Gail received her BS degree in physics, with an emphasis in optics, from San Diego State University in San Diego, CA in May 1986.