SOLID-STATE CHILLERS: Waveguides, lenses keep optical refrigerator very cold
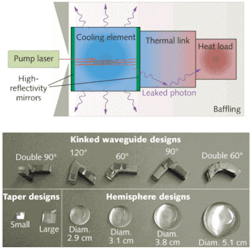
Back in 1995, Laser Focus World reported on a developing optical refrigeration technique from Los Alamos National Laboratory (Los Alamos, NM) in which 1020 nm light from an ytterbium fiber laser is beamed into a rare-earth-doped, heavy-metal fluoride (ZBLANP) glass. The material absorbs this light and causes it to emit higher-energy photons, effectively cooling the glass and creating a solid-state chiller in a process called anti-Stokes fluorescence. Although by 1999 this initial work resulted in a cooling drop of 65°C (to 208 K) within the material, researchers at Los Alamos National Laboratory and Harvey Mudd College (Claremont, CA) have improved on this work by using optical waveguides and other lensing materials to achieve a cooling drop of 166°C (107 K).1
For practical applications, a cooling element must be thermally connected to some heat load to be cooled. When a 1 cm3 piece of bulk ZBLANP glass is used as the cooling element and pumped by a laser, high-energy-fluorescence photons are emitted isotropically. Dielectric mirrors on both sides of the cooling element typically reflect photons with 0° to 30° angles of incidence; however, rays with higher incident angles leak through the mirrors and are absorbed by the heat load. Unless these photons are directed out of the cooler onto some medium (called baffling) outside the ZBLANP glass, absorption occurs at the heat load and the refrigeration effect is nullified.
For this reason, optical-refrigerator design must include a thermal link to divert high-angle fluorescence photons. This thermal link can then be coupled to a heat load to transfer heat energy to the cooling element while avoiding fluorescent-photon absorption.
Improving thermal-link design
To improve the efficiency of the optical refrigerator, the research team studied several variations of thermal-link designs (see figure).
The first variation used multiple mirrors—highly reflective coatings in the space between the cooling element and the thermal link to reflect light outward onto the baffling. However, a disadvantage of this design is that multiple reflective surfaces can lead to trapped rays that could dramatically reduce the cooling process. A second variation uses a kinked waveguide as the thermal link to essentially place the heat load at a right angle (or off-axis) to the cooling element. A third hemispherical design with a radius larger than the size of the emitting surface from the cooling element limits trapped rays, insuring that high-angle rays exiting the cooling material also exit the thermal link before reaching the heat load. Finally, a fourth thermal link uses a pyramidal taper that decreases the angle of incidence of the rays upon each internal reflection to a point where they can then exit the thermal link at near-normal incidence.
The researchers used a simplified surrogate system to provide an equivalent light source without cooling to compare the light intensity at the location of an absorptive heat load. Although all link designs reduced photon transmission to the thermal load, the most effective thermal link was a 120° kinked waveguide. Tapered designs also performed well.
Thermal performance was calculated using Multiphysics software by Comsol (Burlington, MA). Results indicated that the use of a tapered link result in superior cooling. These models indicated that the current system could potentially chill to 107 K using a very high-power 100 W laser.
Optical performance of the link designs was also modeled using Code V software from Optical Research Associates (Pasadena, CA). While light-intensity values for the various designs were different from experimental values, the order of the designs from best-through-worst performance was consistent.
Harvey Mudd researcher Steven Von der Porten says, “Optical refrigeration won’t likely be cooling your soda, but it does have the potential to achieve cryogenic temperatures in a compact form. Due to the optical nature of the process, it avoids vibrations and the use of consumables—inherent problems with techniques such as liquid cooling.”
REFERENCE
- J. Parker et al., J. Appl.Phys. 105, p. 013116 (Jan. 14, 2009).
About the Author
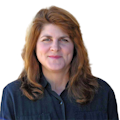
Gail Overton
Senior Editor (2004-2020)
Gail has more than 30 years of engineering, marketing, product management, and editorial experience in the photonics and optical communications industry. Before joining the staff at Laser Focus World in 2004, she held many product management and product marketing roles in the fiber-optics industry, most notably at Hughes (El Segundo, CA), GTE Labs (Waltham, MA), Corning (Corning, NY), Photon Kinetics (Beaverton, OR), and Newport Corporation (Irvine, CA). During her marketing career, Gail published articles in WDM Solutions and Sensors magazine and traveled internationally to conduct product and sales training. Gail received her BS degree in physics, with an emphasis in optics, from San Diego State University in San Diego, CA in May 1986.