PHOTONIC FRONTIERS: MONSTER GROUND TELESCOPES: Bigger is better for ground telescopes: Super-giants will reach 24 to 42 m
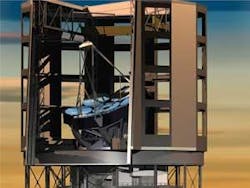
The super-giant telescopes are coming. Three massive ground-based telescopes are being designed with primary mirrors 24 to 42 m in diameter, a huge step beyond today’s biggest instruments. They differ in detail, but share important features, including segmented mirrors, sophisticated control systems, and adaptive optic systems promising resolution better than the Hubble Space Telescope. All aim at beginning observations in 2018, to work with the James Webb Space Telescope.
Why stay on the ground when no telescope on Earth can match Hubble’s most spectacular panoramas of distant galaxies and the jet-black sky of deep space? We can’t afford not to. The least of the giants can collect 100 times more light than Hubble at a fraction of the cost. New technology helps us see more clearly through the atmosphere than ever before. And it doesn’t take years of planning, a space walk, and hundreds of millions of dollars to replace a faulty circuit board.
Generations of giant telescopes
For most of the past 60 years, the 200 in. (5.1 m) Hale Telescope at Palomar Observatory (Palomar Mountain, CA) was the world’s premier telescope. That changed in 1993 after the first Hubble repair mission and completion of the segmented-mirror 10 m Keck-1 telescope at Mauna Kea Observatory in Hawaii. Completion of a duplicate, Keck-2, in 1996 allowed optical interferometry.
Now the three super-giants promise to dwarf the Keck twins. The Giant Magellan Telescope, to be built at Las Campanas Observatory in Chile, will have a 24 m primary assembled from seven 8.4 m mirrors. This month developers will pick a site for the Thirty-Meter Telescope to be built around a 30 m primary consisting of 492 hexagonal mirrors measuring 1.44 m each. The European Southern Observatory (ESO, Garching, Germany) has even bigger plans—the 42 m European Extremely Large Telescope, assembled from 984 hexagonal segments.
Giant Magellan Telescope
The Giant Magellan Telescope is the brainchild of the Carnegie Institution for Science (Washington, D.C.), which is transferring control to a nine-member consortium called GMTO Corp. (Pasadena, CA). The project is expected to cost $600 million.
The 24 m primary will be built from 8.4 m honeycomb-structure disks of borosilicate glass made at the University of Arizona's Steward Observatory Mirror Lab (Tucson, AZ). Gaps between the circular segments will reduce the collecting area to that of a 21.4 m disk, more than four times that of Keck-1.
The large segments offer big advantages, says GMT project manager Matt Johns. “We know how to make them, we have polishing, and we know how to control thermal effects,” says Johns. The big challenge is finishing the six outer mirrors to the precise aspheric shapes needed to assemble the primary. To test the technology, the mirror lab cast a prototype outer disk in 2005 and has nearly finished figuring it. Exhaustive testing will follow a year of polishing starting this summer. Targets are surface accuracy within 25 nm and focal lengths uniform to within 0.5 mm.
The concave secondary mirror will include seven 1.1 m disks with thin adaptive-optic surfaces for wavefront control, each collecting light from one primary segment. The telescope is a classic Gregorian, focusing light through a central hole in the primary (see Fig. 1). A fixed compensating lens will correct for mirror aberrations, and yield a visible field of view of 20 arcmin. Two adaptive-optics systems will operate in the infrared. A laser guide star system will compensate for perturbations from the ground to the upper atmosphere across a 30 arcsec field. A ground-layer adaptive-optics system will compensate only for low-level turbulence, but will offer an 8 arcmin field. The designers hope to reach resolution 10 times finer than the 2.4 m Hubble.
An 18 m focal length will make the primary very fast, f/0.7, but the secondary will extend the overall focal length to 202.74 m, or f/8.4, with an image scale of 1 arcsec per millimeter. Large instruments will be mounted in a 6 × 5 m volume behind the center of the primary. Flat folding mirrors will serve smaller instruments. The whole moving assembly will weigh just over 1000 tons.
The Thirty-Meter Telescope
The Association of Canadian Universities for Research in Astronomy, the California Institute of Technology (Pasadena) and the University of California began planning the Thirty-Meter Telescope (TMT) in 2003. In June they held a preliminary design review for the $1 billion telescope. This month the TMT board will decide whether to build on Mauna Kea or at Cerro Armazones, a new site in Chile.Design of the 30 m primary assembled from 492 hexagonal 1.44 m segments draws heavily on technology developed for Keck, says TMT optics group leader Eric Williams. “Keck pioneered the ability to control mirror segments and phase up the array to make it act as one optical surface,” says Williams. The real-time control system will move three actuators on each segment up to 20 times a second to correct for wind, thermal, and mechanical disturbances, and keep segments aligned within 5 nm. Gaps are only 2.5 mm.
The TMT designers picked segments smaller than the 1.8 m used on Keck because the size is more widely available from suppliers and would encourage competition. “We think this is the sweet spot,” says Williams, and ESO has chosen the same size for its super-giant. The f/1 hyperboloid primary of TMT will require 75 tons of glass, with 82 different surface profiles for the segments.
The wide-field folded Ritchey-Chrétien design will have a 3.1 m monolithic convex secondary (see Fig. 2). The tertiary mirror is a 2.5 × 3.5 m flat aligned at 45° to direct light to Nasmyth focuses on the sides. The primary mirror pivots on a line connecting the two Nasmyth platforms, which rotate around the same vertical axis as the primary. The f/15 system forms an image 20 m from the center of the tertiary mirror.
In addition to giving near-diffraction-limited resolution, adaptive optics will reduce integration times needed to detect faint point sources against background noise, says Brent Ellerbroek, leader of the TMT adaptive-optics group. Increasing telescope size alone reduces integration time by the diameter squared. But adaptive optics reduces background and concentrates light, reducing integration time by the fourth power of diameter.
Ellerbroek’s group plans to create an array of laser guide stars by tuning six 25 W lasers to the 589 nm D2 line of sodium in the upper atmosphere. He says the array should compensate for turbulence in a larger volume of the atmosphere than the line to a conventional single guide star. Candidates for the laser source are sum-frequency generation from the 1064 and 1339 nm lines of Nd:YAG, and frequency doubling of a 1178 nm fiber-Raman laser. He expects the guide-star array to reduce root-mean-square wavefront error to 200 nm yielding near-diffraction-limited images across a 30 arcsec field from 1.25 to 2.2 µm. The entire telescope’s field of view will be 15 to 20 arcmin.The European Extremely Large Telescope
The biggest super-giant under development is the ESO’s 42 m European Extremely Large Telescope (E-ELT). Selecting the same hexagonal segment size as TMT “will help us to have a credible production facility in industry,” says Colin Cunningham of the U.K. Astronomy Technology Centre at the Royal Observatory Edinburgh (Edinburgh, Scotland). But with twice the area, E-ELT will require 984 segments and cost around €850 million ($1.15 billion). Instrumentation will add another €150 million ($200 million).
The E-ELT uses a novel five-mirror design that gives better optical correction than is possible with three mirrors, at the cost of higher scattering and thermal emission. A large secondary, up to 6 m across, will focus light onto a 4.2 m tertiary mirror, which will relay the light to a 2.6 m adaptive mirror with about 8000 actuators adjusting its shape 1000 times a second to compensate for atmospheric turbulence. A final 2.7 m mirror will clean up optical imperfections and deliver light to folding mirrors or instruments.
Like TMT, E-ELT will deliver light to instruments at the Nasmyth foci on the sides of the primary mirror, where the field of view will be 10 to 20 arcmin, depending on the optical arrangement. The large deformable mirror will compensate for ground-layer air turbulence, giving a working field of about 5 arcmin for general science observations. A separate instrument is in development for compensation for turbulence over longer paths in small areas of the sky. The idea is to individually correct the light from up to 20 objects, using separate deformable mirrors.
The ESO held an intermediate design review in May, with a detailed design due in June 2010. Developers are looking at several potential sites, including two in Chile, and one each in the Argentine Andes, the Canary Islands, and Morocco.
Outlook
Developers face major engineering challenges in building super-giant telescopes. The optical and mechanical issues involved in doubling to quadrupling telescope diameters are formidable. So are the challenges in improving adaptive optics to take full advantage of gigantic apertures.
However, the biggest challenges will be financial. All three groups have committed serious money to their projects, and are well along the road to final designs. All plan to start operations in 2018. Ground-based telescopes look almost cheap compared to orbiting observatories like Hubble and Webb. Nonetheless, all of the developers must raise considerably more money to finish their telescopes, and that’s not going to be easy in the current economic crisis.
Yet the super-giants are awesome, not just in their size but in their scientific potential. “There’s an outside chance we can do a direct observation of an Earth-like planet, and even measure its atmospheric spectrum,” says Cunningham. With their huge light-gathering capabilities, the super-giants could crack mysteries of the early universe. A decade or two of measuring the velocity of quasars might reveal changes in their velocity, giving us the first direct measurement of the Hubble constant of the expanding universe.
MORE INFORMATION
Giant Magellan Telescope: www.gmto.org
Thirty-Meter Telescope: www.tmt.org
European Extremely Large Telescope: www.eso.org/public/astronomy/projects/e-elt.html
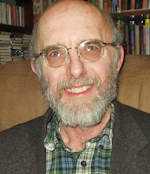
Jeff Hecht | Contributing Editor
Jeff Hecht is a regular contributing editor to Laser Focus World and has been covering the laser industry for 35 years. A prolific book author, Jeff's published works include “Understanding Fiber Optics,” “Understanding Lasers,” “The Laser Guidebook,” and “Beam Weapons: The Next Arms Race.” He also has written books on the histories of lasers and fiber optics, including “City of Light: The Story of Fiber Optics,” and “Beam: The Race to Make the Laser.” Find out more at jeffhecht.com.